2
Chemoresistance in Ovarian Cancer: The Role of Malignant Ascites
Mariana Nunes1,2 • Sara Ricardo1,3,4
1Differentiation and Cancer Group, Institute for Research and Innovation in Health (i3S) of the University of Porto, Porto, Portugal/Institute of Molecular Pathology and Immunology, University of Porto (IPATIMUP), Porto, Portugal; 2School of Medicine and Biomedical Sciences (ICBAS), University of Porto, Porto, Portugal; 3Faculty of Medicine, University of Porto (FMUP), Porto, Portugal; 4Toxicology Research Unit (TOXRUN), University Institute of Health Sciences (CESPU), Gandra, Portugal
Abstract: Ascites is an inflammatory process that induces the abnormal accumulation of a large amount of fluid into the peritoneal cavity. This pathological condition is observed in many neoplasms harboring peritoneal dissemination, a common feature in advanced ovarian cancer. In almost all patients, recurrent disease is accompanied by the accumulation of malignant ascites and is associated with chemoresistance and poor prognosis. The malignant ascites comprises a reservoir of a complex mixture of cellular components and soluble factors which provides a pro-inflammatory and tumor-promoting microenvironment for cancer cells. Moreover, tumor cells exhibit cancer stem-like phenotypes, acquire enhanced resistance to therapies, and higher capacity for metastatic spread and recurrent disease. The accessibility to malignant ascites and its cellular components makes it a unique source to track tumor progression and a key element to overcome chemoresistance.
Keywords: chemoresistance in ovarian cancer; malignant ascites in ovarian cancer; patient-derived organoids; personalized medicine in ovarian cancer; transcoelomic dissemination in ovarian cancer
Author for correspondence: Sara Ricardo, Differentiation and Cancer Group, Institute for Research and Innovation in Health (i3S) of the University of Porto, Porto, Portugal/Institute of Molecular Pathology and Immunology, University of Porto (IPATIMUP), Porto, Portugal. E-mail: sricardo@ipatimup.pt
Cite this chapter as: Nunes M, Ricardo S. Chemoresistance in Ovarian Cancer: The Role of Malignant Ascites. In: Lele S. editor. Ovarian Cancer. Brisbane (AU): Exon Publications. Online first 01 Aug 2022.
Doi: https://doi.org/10.36255/exon-publications-ovarian-cancer-malignant-ascites
In: Lele S (Editor). Ovarian Cancer. Department of Gynecologic Oncology, Roswell Park Comprehensive Cancer Center, Buffalo, New York, USA. ISBN: 978-0-6453320-8-7. Doi: https://doi.org/10.36255/exon-publications-ovarian-cancer
Copyright: The Authors.
License: This open access article is licenced under Creative Commons Attribution-NonCommercial 4.0 International (CC BY-NC 4.0) https://creativecommons.org/licenses/by-nc/4.0/
INTRODUCTION
Ascites can occur in different diseases, including cirrhosis, pancreatitis, nephritis, heart failure, and cancer (1, 2). Malignant ascites (MA) refers to a pathological accumulation of fluid into the peritoneal cavity, being present in several neoplasms e.g., ovarian, endometrial, pancreatic, gastric, colorectal, liver, and peritoneal malignancies (1, 3–5). Also, ascites can occur at a lower frequency in extra-abdominal tumors, e.g., lung and breast cancers (3, 4). This inflammatory condition occurs as a disruption in the balance of fluid production and reabsorption (4, 6) by different pathophysiologic mechanisms including increased vascular permeability––largely driven by upregulation of vascular endothelial growth factor (VEGF)––peritoneal lymphatic obstruction, and high levels of fluid production (3, 4, 7, 8). The presence of MA is often indicative of tumor cells in peritoneal cavity or peritoneal carcinomatosis (7) and can cause several comorbidities e.g., dyspnea, abdominal tenderness and painfulness, nausea, anorexia, fatigue, early satiety, weight change, and compromised movements (3, 9).
MA is considered a hallmark in advanced ovarian cancer as more than one-third of the patients develop this condition (6, 9, 10) and occurs in all epithelial ovarian cancer subtypes, including serous [i.e., low-grade and high-grade serous carcinomas (HGSC)], clear cell, mucinous, and endometrioid carcinomas (10, 11). MA accumulation is significantly higher in HGSC, the most aggressive subtype (10, 12).
MA containing a variety of cellular and acellular components associated with poor prognosis, provides a nurturing environment for cancer progression, metastasis, chemoresistance, and recurrence (Figure 1A) (3–6, 13). Also, the immunological constituents of MA enhance an inflammatory environment through the secretion of pro-inflammatory cytokines and chemokines accelerating disease progression (6, 13). Chemotherapeutic agents can prevent MA accumulation, however, chemoresistant or recurrent disease commonly develop intractable ascites that lead to a worse prognosis (4, 6). A persistent accumulation implies a repeated paracentesis for palliation, however, this temporary solution to relieve symptoms may lead to clinical complications, such as catheter-associated infections (3, 6, 13). Nevertheless, this regularity allows sampling cellular and acellular components from MA during tumoral progression, providing a unique opportunity for translational research (5, 14).
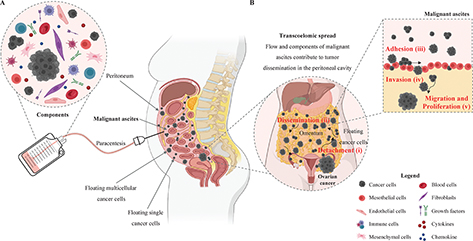
Figure 1 The accumulation of malignant ascites in ovarian cancer. A, Malignant ascites comprising cancer and non-cancer cells (e.g., fibroblasts, mesothelial, endothelial, immune, mesenchymal and blood cells) and acellular components (e.g., growth factors, cytokines, and chemokines). B, Malignant ascites contributes to transcoelomic dissemination, i.e., ovarian cancer cells detach from primary tumor in single or multicellular spheroids (i) and travel to other peritoneal sites (ii). Ovarian cancer cells adhere and implant on the peritoneum and peritoneal organs surface (e.g., omentum), where they clear the mesothelial lining (iii), invade the submesothelial extracellular matrix (iv), migrate, and proliferate creating secondary lesions (v). MA, malignant ascites; OC, ovarian cancer.
TRANSCOELOMIC DISSEMINATION
The most common route of dissemination in ovarian cancer is the transcoelomic spreading across the peritoneal cavity (15, 16), but, less commonly, can also occur by lymphatic and hematogenous spreading (6, 15). Transcoelomic dissemination leads to peritoneal carcinomatosis, a more diffuse and widespread metastatic form that have a high negative impact in surgical resectability (17). This metastatic route is a more efficient process for cancer spread since tumor cells follow the dynamics of peritoneal fluid until they meet the mesothelial lining, where they implant and aggregate (8). Metastatic implants are dispersed in areas with constant and extensive contact to peritoneal fluid, especially the omentum, Pouch of Douglas and right subphrenic region (18, 19). In ovarian cancer, peritoneal metastasis involves shedding of cells from primary tumor, dissemination in the abdominal cavity, attachment and invasion of the mesothelial lining, and colonization of intra-peritoneal organs (Figure 1B) (16, 20). Indeed, MA is described as a requirement for transcoelomic metastasis facilitating the dissemination of tumor cells and acting as a growth-promoting environment (20, 21) and is also reported as a promotor of lymphatic and hematogenous dissemination (22).
THE MICROENVIRONMENT OF MALIGNANT ASCITES
MA is composed of tumor and non-tumor cells (e.g., fibroblasts, adipocytes, mesothelial, endothelial, and inflammatory cells) (6, 23) and a liquid acellular fraction, all contributing to tumor progression, metastasis, and chemoresistance (7, 24). Frequently, MA contain cell aggregates (both cancer and non-cancer cells) forming spheroids that have a higher metastatic potential (25, 26). Several in vitro multicellular spheroids systems present anoikis resistance (27), restricted access and limited efficacy of cytotoxic drugs (25, 28) and slowly cycling and quiescent states becoming more chemoresistant (29, 30). Lafiti et al. showed that 95% of chemoresistant patients had an increased proportion of spheroids in its MA when compared to chemo-naïve (25%) (26). Moreover, MA liquid fraction contain tumor-derived circulating free DNA (cfDNA) that presents an opening for tracking changes in the mutational profiles that cause chemoresistance and relapse (5).
The acellular components of MA involve a dynamic reservoir of pro- and anti-tumorigenic factors, comprising cytokines (31–35), chemokines, growth factors (36), integrins (37, 38) and metabolites contributing to metastasis and chemoresistance (5, 23, 28). In ovarian cancer, the cytokine profiles of MA demonstrated high levels of pro-tumorigenic (e.g., interleukin (IL)-6, IL-8, IL-10 and IL-15) and reduced levels of anti-tumorigenic (e.g., IL-2, IL-5, IL-7 and IL-17) factors contributing to a pro-inflammatory and immunosuppressive tumor microenvironment (4, 23, 34, 39). IL-6 and IL-10 are both correlated with poor prognosis and reduced therapy response (32, 40). High levels of IL-6 promotes MA accumulation, and ovarian cancer progression (40) and dissemination (41, 42). It is also associated with shorter progression free survival (PFS) (40, 43), poor overall survival (OS), reduced initial therapy response, and development of chemoresistance (35).
In ovarian cancer, high levels of VEGF are correlated with MA accumulation (36, 44) and poor prognosis (45). Zhan et al. showed that MA has high levels of VEGF compared to benign ascites and is associated with poor survival rates (36). Also, VEGF is commonly related with advanced-stage disease, high tumor grade, and increased metastatic potential (7). In ovarian cancer tissues, high epidermal growth factor receptor (EGFR) expression is often associated with aggressive, invasive, and metastatic disease (46, 47) and decreased OS and disease-free survival (48). Extracellular vesicles comprise another class of factors abundantly expressed in MA being important mediators of crosstalk between cancer cells and their microenvironment (49, 50). A recent study demonstrated that extracellular vesicles containing glycolytic pathway-related proteins can transmit chemoresistance to other tumor cells, facilitating disease progression (51). The proteomic profiling of MA enables the identification of possible therapeutic options to overcome ovarian cancer chemoresistance (52–55). Based on high-resolution mass spectrometry analysis, Ahmed et al. identified differential expression of 178 diminished, and 175 enriched proteins in MA of chemoresistant ovarian cancer patients compared to MA of chemonaïve patients (52).
Both cellular and acellular components display crucial roles in regulating proliferation, metastasis, and chemoresistance. Hence, exploring MA cell populations and signaling molecules during disease progression, and specially over the course of therapy (e.g., comparing patients in remission versus relapse), will be crucial to improve patient outcomes (5).
THE MANAGEMENT OF MALIGNANT ASCITES AND CHEMORESISTANCE
Over the last decades, the standard care for advanced ovarian cancer patients is based on platinum (e.g., Carboplatin) and taxane-based (e.g., Paclitaxel) chemotherapy following cytoreductive surgery (56, 57). In some cases, neoadjuvant chemotherapy is performed as alternative to standard treatment procedures (58, 59). The management of MA can be performed by aspiration during debulking surgery, chemotherapeutic schemes, or a paracentesis procedure (i.e., removing MA inserting a wide-bore needle percutaneously through abdominal wall) (5, 7). Paracentesis is the most frequent procedure providing immediate relief symptoms, however, requires repeated drawings and can cause serious comorbidities and complications, such as draining site continuous leakage or bowel perforation (4, 60, 61). Most of the chemoresistant patients present intractable MA being submitted to repeated paracentesis during its’ clinical course (4, 7).
Drainage catheter is placed percutaneously in the peritoneal cavity is an alternative intervention for MA management, being easy to self-drain, increasing patient independence, and diminishing the necessity for constant paracentesis at the hospital (62). It has been shown that intraperitoneal (IP) catheters drainage is a successful procedure and have a low complication rates supporting their use as first-line approach in refractory MA (63). Recently, Fotopoulou et al. demonstrated that in patients with hepatic and ovarian malignancies, subcutaneously implanted Sequana Medical Alfapump System, which continuously drains MA via urinary bladder, reduced the number of paracenteses from 4 to 1 (64, 65). However, more studies are essential to include catheter drainage as a routine procedure in oncological practices.
IP chemotherapy, i.e., the administration of high chemotherapy concentrations in the peritoneal cavity, directly exposes of peritoneal organs surfaces to chemotherapy agents compared to intravenous management and minimizes the toxicity of systemic chemotherapy (3, 4). A study performed in ovarian cancer patients at stage III with optimally debulked showed that IP administration as first-line treatment had a 16-month survival benefit (66). However, less than half of women completed all chemotherapy cycles, and it was shown a higher complication and toxicity rates in these patients (66). Early phase trials studying chemotherapy to control MA indicate that IP is the most effective method to deliver chemotherapy (4). Hyperthermic intraperitoneal chemotherapy (HIPEC), a combination of cytoreductive surgery and high concentration of heated chemotherapeutic agents, can reduce MA (67), since cytoreductive surgery removes macroscopic tumor implants, and IP act directly in the peritoneal cavity improving drug absorption and efficacy, targeting the remaining microscopic tumoral implants (68, 69). In advanced ovarian cancer with extensive peritoneal metastasis, HIPEC can be effective in improving patient survival (70, 71). Van Driel et al. showed in stage III ovarian cancer patients that combining cytoreductive surgery with HIPEC resulted in longer OS and PFS compared to surgery alone (72). Nevertheless, it is difficult to include HIPEC as a standard treatment as several studies demonstrate significant variation in patient outcome, as they use different chemotherapeutic drugs, concentrations, temperatures, and procedures duration leading to heterogenous and incomparable studies (70, 73). Thus, we need more well-designed trials to reach a more specific conclusion regarding the use of IP and HIPEC as a crucial armamentarium for ovarian cancer.
Studies focused on pharmacological therapy to MA management are limited and include antiangiogenic and metalloproteinase (MMP) inhibitors, and immunological modulators (4, 74–77). Bevacizumab is an anti-angiogenic targeting VEGF that delays the recurrent disease and palliate symptoms associated with MA accumulation (4, 78, 79). Numnum et al. reported that Bevacizumab treatment reduced the levels of MA allowing the discontinuation of repeated paracentesis (78). Several phase III trials in ovarian cancer, e.g., GOG18 (NCT00262847) and ICON7 (NCT00483782) added Bevacizumab to standard treatments and reported improved PFS and OS in patients with advanced stage disease (80, 81). Also, the randomized clinical trials OCEANS (NCT00434642) and AURELIA (NCT00976911) indicate that Bevacizumab can provide benefits, delaying the recurrences in platinum-sensitive and platinum-resistant ovarian cancer (82, 83). In previously mentioned trials, Bevacizumab was not introduced with primary intention of affecting MA but revealed very effective in the reduction of its’ formation (84, 85). Recently, a study demonstrated that IP administration of Bevacizumab present an acceptable safety profile and reduces the formation or delay MA accumulation in chemoresistant patients (86). A pilot study using Aflibercept (VEGF inhibitor) showed that this drug increased the time between paracentesis (87). Moreover, preclinical models demonstrated that Aflibercept prevent MA accumulation and inhibits tumor proliferation through VEGF blockade, however, it was observed an increased risk of morbidity associated with bowel perforation (75, 85). Batimastat (MMP inhibitor) has been used in animal models studies and demonstrated to decrease tumor growth, metastasis, and MA volume (88, 89). However, a Phase I study that administered Batimastat intraperitoneally in ovarian cancer patients found that the decreased MA volume was limited to a small number of patients (90, 91). Using an ovarian cancer mouse model, Zhao et al. demonstrated that Losartan (antihypertensive therapy targeting renin-angiotensin system) as a matrix-depleting strategy enhances the efficacy of Paclitaxel and reduces MA (92). Octreotide (Somatostatin analogue) is particularly useful for chylous ascites (a rare form containing large amounts of triglycerides), reducing splanchnic blood flow, contributing to a decreased lymph flow and bowel obstructive symptoms (7). Jatoi et al. revealed that a monthly intramuscular injections of long-acting Octreotide delayed the need for paracentesis from 14 to 28 days (93). The efficacy of Batimastat, Losartan and Octreotide is still under scrutiny and more studies are needed to clarify is role in MA management.
Several studies suggest improvements in the management of MA using immunological approaches including IP triamcinolone (76), IP interferon α and β (94), tumor necrosis factor (95) and even non-pathogenic infectious agents (96, 97). Recently, a prospective randomized phase II/III trials were conducted in ovarian cancer patients with MA using Catumaxomab, a trifunctional IgG2 antibody (anti-CD3, anti-EpCAM and anti-Fc receptors) that generates a strong immune reaction response against tumor cells (4, 61, 77). Heiss et al. demonstrated that IP administration of Catumaxomab leads to longer paracentesis-free survival, fewer ascites-related symptoms, and improved palliation (77). In 2009, Catumaxomab became the first therapeutic agent approved for MA management in Europe (4, 77).
Summing up, the previously described treatment options revealed a limited success in the management of MA in ovarian cancer patients. It is crucial to test more effective drugs and to develop improved methods for drug delivery to prevent MA accumulation during the ovarian cancer patient’s clinical course.
MALIGNANT ASCITES AS AN OPPORTUNITY FOR TRANSLATION RESEARCH
MA is an exceptionally good source for research due to easy accessibility, repeated collection, capacity for reflecting primary tumor, metastatic implants, and tumor microenvironments. MA enables successive sampling of the milieu and therefore, an ideal resource for translational studies including prediction of drug response and monitoring drug efficacy (4, 5, 98). MA accumulation is more frequently observed in recurrent cases and is absent in cases where patients respond to therapy (5). The understanding of the mechanisms involved in accumulation of MA and metastatic spread of ovarian cancer will allow the identification of potential drug targets that could be used to overcome chemoresistance. The pathways responsible for driving EMT phenotypic changes are responsible for spheroids tumorigenicity and can be potential targets (5, 99). Saine et al. reported that high expression levels of signal transducer and activator of transcription 3 (STAT3) in MA-derived ovarian cancer cells promote invasion and metastasis (100). In mouse models, it was shown that STAT3 inhibitors reduced chemoresistance and spheroid tumorigenicity (100, 101). Other studies showed that transforming growth factor (TGF)-β present in MA cells is a major driver in metastatic spread (99, 102). Acellular components provide a microenvironment that sustain cancer cells survival and potentiate the discovery of new treatment strategies based on disrupting this tumoral environment (103). By analyzing malignant effusions from ovarian cancer patients, Davidson et al. demonstrated high expression levels of AKT, cAMP-responsive element binding protein (CREB) and JUN N-terminal kinase (JNK) compared to benign effusions (104). Also, high levels of p8, and an increased ratio of phosphorylated EGFR and phosphorylated JNK were associated with worse outcome (104). Thus, all mentioned proteins and respective pathways are potential therapeutic targets for overcoming chemoresistance and the abrogation of peritoneal metastization of ovarian cancer.
A PERSONALIZED MEDICINE APPROACH FOR OVARIAN CANCER USING MALIGNANT ASCITES
The frequency of MA occurrence at first presentation, and subsequent relapse in ovarian cancer, provides a highly accessible pool of biologic material to track the sensitivity or resistance of tumor cells, as it captures several populations that compose the tumor microenvironment (4, 5). Serial samples, e.g., pre- and pos-chemotherapy could be compared to assess molecular changes that may be predictive of therapeutic responses (103–105).
Conventional treatment strategies just allow a “one-size-fits-all” treatment based on a limited panel of drugs excluding alternative opportunities (106). The use of patient-derived tumor cells allows the association of specific tumor characteristics with a personalized treatment (106). Recently, patient-derived organoids (PDOs) emerged as a powerful modeling approach in cancer research (107–110) as many studies, in different cancer models, established PDOs that recapitulate the features from original derived lesions (111–116). Recently, Velletri et al. used ovarian cancer clinical samples across primary tumours and metastatic sites and demonstrated that MA-derived organoids retain key subpopulations and recapitulate features of the original samples acting as ‘patient-matched avatars’ that can be used in a precision oncology platform (117, 118). Moreover, other groups have demonstrated the capacity for growing tumor cells from MA in vitro for drug sensitivity testing (106, 119) and predicting clinical responses to therapy through assessment of biomarkers present in MA tumor cells (32, 120, 121). A study by Bi et al. demonstrated that PDOs can be established in a high percentage of cases to perform drug tests in a timely manner and that PDOs have the potential to identify more efficient regiments (106). In addition, they demonstrated a specific case in which the PDO revealed patients resistance to standard therapy (106). Another study showed that the exposure of MA-derived spheroids to a panel of drugs can reflect the patients’ therapy responses and identify the best viable candidates (122).
Many co-clinical trials (i.e., preclinical studies and clinical trials are conducted in parallel) are currently underway in different tumoral contexts, including ovarian cancer, comparing therapy responses in PDOs to corresponding patient outcomes, e.g., NCT04555473 and NCT05175326. This new strategy enables real time data integration to accurately stratify and customize treatment of patients (123). Additional clinical trials propose to evaluate therapeutical responses in PDOs to predict the clinical drug efficacy and choose the best regimen for each patient to guide clinical decisions, e.g., NCT04279509 and NCT04768270.
The establishment of PDOs from MA offers a valuable preclinical platform since they can be obtained with high efficiency in a short-period time, since MA contain cellular aggregates that are “natural” PDOs floating in the ascitic fluid (124). These systems can be used in drug efficacy tests using a range of approved and novel compounds (single or combined regiment) in a case-specific and in an acceptable time frame to predict therapy responses and guiding clinical decisions (Figure 2) (106, 125–128).
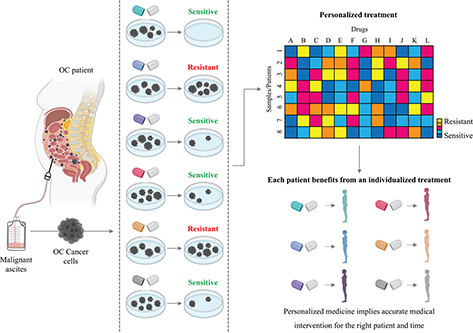
Figure 2 A personalized medicine approach in ovarian cancer using malignant ascites. Malignant ascites obtained from ovarian cancer patients by paracentesis will be used to make PDOs models and exposed to a panel of drugs to predict patients’ responses. The most promising drugs will be selected to be administrated in the corresponding patient. MA, malignant ascites; OC, ovarian cancer; PDOs, patient-derived organoids.
CONCLUSION
Currently, therapeutic options for MA management generate initial acceptable responses but the efficacy in very low in the long-term. The accumulation of MA embodies the poorest outcomes representing a significant clinical challenge for ovarian cancer management. However, MA constitutes a unique opportunity for translational research. Large volumes of MA can be removed from patients, often repeatedly, representing a successive sampling of the tumor milieu in which ovarian cancer spreads making this an ideal source of biologic material to monitor chemoresistance and test several therapeutical options ex-vivo.
Acknowledgement: This work was developed at i3S/IPATIMUP, an Associate Laboratory of the Ministério da Ciência, Tecnologia e Ensino Superior (MCTES), and partially supported by Fundação para a Ciência e a Tecnologia (FCT)/ MCTES. Mariana Nunes acknowledges FCT/ MCTES and União Europeuia (UE) for financial support through a PhD fellowship (2020.04720.BD) co-sponsored by Fundo Social Europeu (FSE) through Programa Operacional Regional Norte (Norte 2020).
Conflict of Interest: The authors declare no potential conflict of interest with respect to research, authorship and/or publication of this chapter.
Copyright and Permission Statement: The authors confirm that the materials included in this chapter do not violate copyright laws. Where relevant, appropriate permissions have been obtained from the original copyright holder(s), and all original sources have been appropriately acknowledged or referenced.
REFERENCES
- Sangisetty SL, Miner TJ. Malignant ascites: A review of prognostic factors, pathophysiology and therapeutic measures. World J Gastrointest Surg. 2012;4(4):87-95. https://doi.org/10.4240/wjgs.v4.i4.87
- Chiejina M, Kudaravalli P, Samant H. Ascites. StatPearls. Treasure Island (FL)2022.
- Cavazzoni E, Bugiantella W, Graziosi L, Franceschini MS, Donini A. Malignant ascites: pathophysiology and treatment. Int J Clin Oncol. 2013;18(1):1–9. https://doi.org/10.1007/s10147-012-0396-6
- Kipps E, Tan DS, Kaye SB. Meeting the challenge of ascites in ovarian cancer: new avenues for therapy and research. Nat Rev Cancer. 2013;13(4):273-82. https://doi.org/10.1038/nrc3432
- Ford CE, Werner B, Hacker NF, Warton K. The untapped potential of ascites in ovarian cancer research and treatment. Br J Cancer. 2020;123(1):9-16. https://doi.org/10.1038/s41416-020-0875-x
- Ahmed N, Stenvers KL. Getting to know ovarian cancer ascites: opportunities for targeted therapy-based translational research. Front Oncol. 2013;3:256. https://doi.org/10.3389/fonc.2013.00256
- Rickard BP, Conrad C, Sorrin AJ, Ruhi MK, Reader JC, Huang SA, et al. Malignant Ascites in Ovarian Cancer: Cellular, Acellular, and Biophysical Determinants of Molecular Characteristics and Therapy Response. Cancers (Basel). 2021;13(17). https://doi.org/10.3390/cancers13174318
- Lengyel E. Ovarian cancer development and metastasis. Am J Pathol. 2010;177(3):1053-64. https://doi.org/10.2353/ajpath.2010.100105
- Ayantunde AA, Parsons SL. Pattern and prognostic factors in patients with malignant ascites: a retrospective study. Ann Oncol. 2007;18(5):945–9. https://doi.org/10.1093/annonc/mdl499
- Krugmann J, Schwarz CL, Melcher B, Sterlacci W, Ozalinskaite A, Lermann J, et al. Malignant ascites occurs most often in patients with high-grade serous papillary ovarian cancer at initial diagnosis: a retrospective analysis of 191 women treated at Bayreuth Hospital, 2006-2015. Arch Gynecol Obstet. 2019;299(2):515-23. https://doi.org/10.1007/s00404-018-4952-9
- Szender JB, Emmons T, Belliotti S, Dickson D, Khan A, Morrell K, et al. Impact of ascites volume on clinical outcomes in ovarian cancer: A cohort study. Gynecol Oncol. 2017;146(3):491–7. https://doi.org/10.1016/j.ygyno.2017.06.008
- Grabowski JP, Harter P, Heitz F, Pujade-Lauraine E, Reuss A, Kristensen G, et al. Operability and chemotherapy responsiveness in advanced low-grade serous ovarian cancer. An analysis of the AGO Study Group metadatabase. Gynecol Oncol. 2016;140(3):457-62. https://doi.org/10.1016/j.ygyno.2016.01.022
- Penet MF, Krishnamachary B, Wildes FB, Mironchik Y, Hung CF, Wu TC, et al. Ascites Volumes and the Ovarian Cancer Microenvironment. Front Oncol. 2018;8:595. https://doi.org/10.3389/fonc.2018.00595
- Coleman RL, Monk BJ, Sood AK, Herzog TJ. Latest research and treatment of advanced-stage epithelial ovarian cancer. Nat Rev Clin Oncol. 2013;10(4):211-24. https://doi.org/10.1038/nrclinonc.2013.5
- Tan DS, Agarwal R, Kaye SB. Mechanisms of transcoelomic metastasis in ovarian cancer. Lancet Oncol. 2006;7(11):925-34. https://doi.org/10.1016/S1470-2045(06)70939-1
- Coelho R, Marcos-Silva L, Ricardo S, Ponte F, Costa A, Lopes JM, et al. Peritoneal dissemination of ovarian cancer: role of MUC16-mesothelin interaction and implications for treatment. Expert Rev Anticancer Ther. 2018;18(2):177-86. https://doi.org/10.1080/14737140.2018.1418326
- Coccolini F, Gheza F, Lotti M, Virzi S, Iusco D, Ghermandi C, et al. Peritoneal carcinomatosis. World J Gastroenterol. 2013;19(41):6979-94. https://doi.org/10.3748/wjg.v19.i41.6979
- Meyers MA. Distribution of intra-abdominal malignant seeding: dependency on dynamics of flow of ascitic fluid. Am J Roentgenol Radium Ther Nucl Med. 1973;119(1):198-206. https://doi.org/10.2214/ajr.119.1.198
- Buy JN, Moss AA, Ghossain MA, Sciot C, Malbec L, Vadrot D, et al. Peritoneal implants from ovarian tumors: CT findings. Radiology. 1988;169(3):691–4. https://doi.org/10.1148/radiology.169.3.3186993
- Weidle UH, Birzele F, Kollmorgen G, Rueger R. Mechanisms and Targets Involved in Dissemination of Ovarian Cancer. Cancer Genomics Proteomics. 2016;13(6):407-23. https://doi.org/10.21873/cgp.20004
- Al Habyan S, Kalos C, Szymborski J, McCaffrey L. Multicellular detachment generates metastatic spheroids during intra-abdominal dissemination in epithelial ovarian cancer. Oncogene. 2018;37(37):5127-35. https://doi.org/10.1038/s41388-018-0317-x
- Ayhan A, Gultekin M, Taskiran C, Dursun P, Firat P, Bozdag G, et al. Ascites and epithelial ovarian cancers: a reappraisal with respect to different aspects. Int J Gynecol Cancer. 2007;17(1):68-75. https://doi.org/10.1111/j.1525-1438.2006.00777.x
- Kim S, Kim B, Song YS. Ascites modulates cancer cell behavior, contributing to tumor heterogeneity in ovarian cancer. Cancer Sci. 2016;107(9):1173–8. https://doi.org/10.1111/cas.12987
- Meads MB, Gatenby RA, Dalton WS. Environment-mediated drug resistance: a major contributor to minimal residual disease. Nat Rev Cancer. 2009;9(9):665-74. https://doi.org/10.1038/nrc2714
- Shield K, Ackland ML, Ahmed N, Rice GE. Multicellular spheroids in ovarian cancer metastases: Biology and pathology. Gynecol Oncol. 2009;113(1):143–8. https://doi.org/10.1016/j.ygyno.2008.11.032
- Latifi A, Luwor RB, Bilandzic M, Nazaretian S, Stenvers K, Pyman J, et al. Isolation and characterization of tumor cells from the ascites of ovarian cancer patients: molecular phenotype of chemoresistant ovarian tumors. PLoS One. 2012;7(10):e46858. https://doi.org/10.1371/journal.pone.0046858
- Shield K, Riley C, Quinn MA, Rice GE, Ackland ML, Ahmed N. Alpha2beta1 integrin affects metastatic potential of ovarian carcinoma spheroids by supporting disaggregation and proteolysis. J Carcinog. 2007;6:11. https://doi.org/10.1186/1477-3163-6-11
- Piche A. Malignant peritoneal effusion acting as a tumor environment in ovarian cancer progression: Impact and significance. World J Clin Oncol. 2018;9(8):167-71. https://doi.org/10.5306/wjco.v9.i8.167
- Peart T, Ramos Valdes Y, Correa RJ, Fazio E, Bertrand M, McGee J, et al. Intact LKB1 activity is required for survival of dormant ovarian cancer spheroids. Oncotarget. 2015;6(26):22424-38. https://doi.org/10.18632/oncotarget.4211
- Dong Y, Stephens C, Walpole C, Swedberg JE, Boyle GM, Parsons PG, et al. Paclitaxel resistance and multicellular spheroid formation are induced by kallikrein-related peptidase 4 in serous ovarian cancer cells in an ascites mimicking microenvironment. PLoS One. 2013;8(2):e57056. https://doi.org/10.1371/journal.pone.0057056
- Jia D, Nagaoka Y, Katsumata M, Orsulic S. Inflammation is a key contributor to ovarian cancer cell seeding. Sci Rep. 2018;8(1):12394. https://doi.org/10.1038/s41598-018-30261-8
- Lane D, Matte I, Garde-Granger P, Laplante C, Carignan A, Rancourt C, et al. Inflammation-regulating factors in ascites as predictive biomarkers of drug resistance and progression-free survival in serous epithelial ovarian cancers. BMC Cancer. 2015;15:492. https://doi.org/10.1186/s12885-015-1511-7
- Freedman RS, Deavers M, Liu J, Wang E. Peritoneal inflammation - A microenvironment for Epithelial Ovarian Cancer (EOC). J Transl Med. 2004;2(1):23. https://doi.org/10.1186/1479-5876-2-23
- Matte I, Lane D, Laplante C, Rancourt C, Piche A. Profiling of cytokines in human epithelial ovarian cancer ascites. Am J Cancer Res. 2012;2(5):566-80.
- Penson RT, Kronish K, Duan Z, Feller AJ, Stark P, Cook SE, et al. Cytokines IL-1beta, IL-2, IL-6, IL-8, MCP-1, GM-CSF and TNFalpha in patients with epithelial ovarian cancer and their relationship to treatment with paclitaxel. Int J Gynecol Cancer. 2000;10(1):33-41. https://doi.org/10.1046/j.1525-1438.2000.00003.x
- Zhan N, Dong WG, Wang J. The clinical significance of vascular endothelial growth factor in malignant ascites. Tumour Biol. 2016;37(3):3719-25. https://doi.org/10.1007/s13277-015-4198-0
- Ahmed N, Riley C, Oliva K, Rice G, Quinn M. Ascites induces modulation of alpha6beta1 integrin and urokinase plasminogen activator receptor expression and associated functions in ovarian carcinoma. Br J Cancer. 2005;92(8):1475–85. https://doi.org/10.1038/sj.bjc.6602495
- Hamidi H, Ivaska J. Every step of the way: integrins in cancer progression and metastasis. Nat Rev Cancer. 2018;18(9):533–48. https://doi.org/10.1038/s41568-018-0038-z
- Giuntoli RL, 2nd, Webb TJ, Zoso A, Rogers O, Diaz-Montes TP, Bristow RE, et al. Ovarian cancer-associated ascites demonstrates altered immune environment: implications for antitumor immunity. Anticancer Res. 2009;29(8):2875–84.
- Lane D, Matte I, Rancourt C, Piche A. Prognostic significance of IL-6 and IL-8 ascites levels in ovarian cancer patients. BMC Cancer. 2011;11:210. https://doi.org/10.1186/1471-2407-11-210
- Obata NH, Tamakoshi K, Shibata K, Kikkawa F, Tomoda Y. Effects of interleukin-6 on in vitro cell attachment, migration and invasion of human ovarian carcinoma. Anticancer Res. 1997;17(1A):337–42.
- Nilsson MB, Langley RR, Fidler IJ. Interleukin-6, secreted by human ovarian carcinoma cells, is a potent proangiogenic cytokine. Cancer Res. 2005;65(23):10794–800. https://doi.org/10.1158/0008-5472.CAN-05-0623
- Tempfer C, Zeisler H, Sliutz G, Haeusler G, Hanzal E, Kainz C. Serum evaluation of interleukin 6 in ovarian cancer patients. Gynecol Oncol. 1997;66(1):27–30. https://doi.org/10.1006/gyno.1997.4726
- Masoumi Moghaddam S, Amini A, Morris DL, Pourgholami MH. Significance of vascular endothelial growth factor in growth and peritoneal dissemination of ovarian cancer. Cancer Metastasis Rev. 2012;31(1–2):143–62. https://doi.org/10.1007/s10555-011-9337-5
- Kassim SK, El-Salahy EM, Fayed ST, Helal SA, Helal T, Azzam Eel D, et al. Vascular endothelial growth factor and interleukin-8 are associated with poor prognosis in epithelial ovarian cancer patients. Clin Biochem. 2004;37(5):363–9. https://doi.org/10.1016/j.clinbiochem.2004.01.014
- Zeineldin R, Muller CY, Stack MS, Hudson LG. Targeting the EGF receptor for ovarian cancer therapy. J Oncol. 2010;2010:414676. https://doi.org/10.1155/2010/414676
- Alper O, Bergmann-Leitner ES, Bennett TA, Hacker NF, Stromberg K, Stetler-Stevenson WG. Epidermal growth factor receptor signaling and the invasive phenotype of ovarian carcinoma cells. J Natl Cancer Inst. 2001;93(18):1375–84. https://doi.org/10.1093/jnci/93.18.1375
- Psyrri A, Kassar M, Yu Z, Bamias A, Weinberger PM, Markakis S, et al. Effect of epidermal growth factor receptor expression level on survival in patients with epithelial ovarian cancer. Clin Cancer Res. 2005;11(24 Pt 1):8637–43. https://doi.org/10.1158/1078-0432.CCR-05-1436
- Nakamura K, Sawada K, Kobayashi M, Miyamoto M, Shimizu A, Yamamoto M, et al. Role of the Exosome in Ovarian Cancer Progression and Its Potential as a Therapeutic Target. Cancers (Basel). 2019;11(8). https://doi.org/10.3390/cancers11081147
- Mitra A, Yoshida-Court K, Solley TN, Mikkelson M, Yeung CLA, Nick A, et al. Extracellular vesicles derived from ascitic fluid enhance growth and migration of ovarian cancer cells. Sci Rep. 2021;11(1):9149. https://doi.org/10.1038/s41598-021-88163-1
- Alharbi M, Lai A, Sharma S, Kalita-de Croft P, Godbole N, Campos A, et al. Extracellular Vesicle Transmission of Chemoresistance to Ovarian Cancer Cells Is Associated with Hypoxia-Induced Expression of Glycolytic Pathway Proteins, and Prediction of Epithelial Ovarian Cancer Disease Recurrence. Cancers (Basel). 2021;13(14). https://doi.org/10.3390/cancers13143388
- Ahmed N, Greening D, Samardzija C, Escalona RM, Chen M, Findlay JK, et al. Unique proteome signature of post-chemotherapy ovarian cancer ascites-derived tumor cells. Sci Rep. 2016;6:30061. https://doi.org/10.1038/srep30061
- Kuk C, Kulasingam V, Gunawardana CG, Smith CR, Batruch I, Diamandis EP. Mining the ovarian cancer ascites proteome for potential ovarian cancer biomarkers. Mol Cell Proteomics. 2009;8(4):661–9. https://doi.org/10.1074/mcp.M800313-MCP200
- Shender VO, Pavlyukov MS, Ziganshin RH, Arapidi GP, Kovalchuk SI, Anikanov NA, et al. Proteome-metabolome profiling of ovarian cancer ascites reveals novel components involved in intercellular communication. Mol Cell Proteomics. 2014;13(12):3558–71. https://doi.org/10.1074/mcp.M114.041194
- Elschenbroich S, Ignatchenko V, Clarke B, Kalloger SE, Boutros PC, Gramolini AO, et al. In-depth proteomics of ovarian cancer ascites: combining shotgun proteomics and selected reaction monitoring mass spectrometry. J Proteome Res. 2011;10(5):2286–99. https://doi.org/10.1021/pr1011087
- Manning-Geist BL, Hicks-Courant K, Gockley AA, Clark RM, Del Carmen MG, Growdon WB, et al. Moving beyond “complete surgical resection” and “optimal”: Is low-volume residual disease another option for primary debulking surgery? Gynecol Oncol. 2018;150(2):233–8. https://doi.org/10.1016/j.ygyno.2018.06.015
- Cannistra SA. Cancer of the ovary. N Engl J Med. 2004;351(24):2519–29. https://doi.org/10.1056/NEJMra041842
- Vergote I, Trope CG, Amant F, Kristensen GB, Ehlen T, Johnson N, et al. Neoadjuvant chemotherapy or primary surgery in stage IIIC or IV ovarian cancer. N Engl J Med. 2010;363(10):943–53. https://doi.org/10.1056/NEJMoa0908806
- Kehoe S, Hook J, Nankivell M, Jayson GC, Kitchener H, Lopes T, et al. Primary chemotherapy versus primary surgery for newly diagnosed advanced ovarian cancer (CHORUS): an open-label, randomised, controlled, non-inferiority trial. Lancet. 2015;386(9990):249–57. https://doi.org/10.1016/S0140-6736(14)62223-6
- Becker G, Galandi D, Blum HE. Malignant ascites: systematic review and guideline for treatment. Eur J Cancer. 2006;42(5):589–97. https://doi.org/10.1016/j.ejca.2005.11.018
- Hodge C, Badgwell BD. Palliation of malignant ascites. J Surg Oncol. 2019;120(1):67–73. https://doi.org/10.1002/jso.25453
- Saiz-Mendiguren R, Gomez-Ayechu M, Noguera JJ, Garcia-Lallana A, Marginet C, Cano D, et al. [Permanent tunneled drainage for malignant ascites: initial experience with the PleurX(R) catheter]. Radiologia. 2010;52(6):541–5. https://doi.org/10.1016/j.rx.2010.06.005
- Tapping CR, Ling L, Razack A. PleurX drain use in the management of malignant ascites: safety, complications, long-term patency and factors predictive of success. Br J Radiol. 2012;85(1013):623–8. https://doi.org/10.1259/bjr/24538524
- Fotopoulou C, Berg T, Hausen A, Hennig R, Jalan R, Malago M, et al. Continuous low flow ascites drainage through the urinary bladder via the Alfapump system in palliative patients with malignant ascites. BMC Palliat Care. 2019;18(1):109. https://doi.org/10.1186/s12904-019-0497-3
- Bellot P, Welker MW, Soriano G, von Schaewen M, Appenrodt B, Wiest R, et al. Automated low flow pump system for the treatment of refractory ascites: a multi-center safety and efficacy study. J Hepatol. 2013;58(5):922–7. https://doi.org/10.1016/j.jhep.2012.12.020
- Armstrong DK, Bundy B, Wenzel L, Huang HQ, Baergen R, Lele S, et al. Intraperitoneal cisplatin and paclitaxel in ovarian cancer. N Engl J Med. 2006;354(1):34–43. https://doi.org/10.1056/NEJMoa052985
- de Bree E, Helm CW. Hyperthermic intraperitoneal chemotherapy in ovarian cancer: rationale and clinical data. Expert Rev Anticancer Ther. 2012;12(7):895–911. https://doi.org/10.1586/era.12.72
- Abdel Mageed H, Van Der Speeten K, Sugarbaker P. The many faces of intraperitoneal chemotherapy. Surg Oncol. 2021;40:101676. https://doi.org/10.1016/j.suronc.2021.101676
- Gonzalez-Moreno S, Gonzalez-Bayon LA, Ortega-Perez G. Hyperthermic intraperitoneal chemotherapy: Rationale and technique. World J Gastrointest Oncol. 2010;2(2):68–75. https://doi.org/10.4251/wjgo.v2.i2.68
- Hotouras A, Desai D, Bhan C, Murphy J, Lampe B, Sugarbaker PH. Heated IntraPEritoneal Chemotherapy (HIPEC) for Patients With Recurrent Ovarian Cancer: A Systematic Literature Review. Int J Gynecol Cancer. 2016;26(4):661–70. https://doi.org/10.1097/IGC.0000000000000664
- Cianci S, Riemma G, Ronsini C, De Franciscis P, Torella M, Schiattarella A, et al. Hyperthermic intraperitoneal chemotherapy (HIPEC) for ovarian cancer recurrence: systematic review and meta-analysis. Gland Surg. 2020;9(4):1140–8. https://doi.org/10.21037/gs-20-335
- van Driel WJ, Koole SN, Sikorska K, Schagen van Leeuwen JH, Schreuder HWR, Hermans RHM, et al. Hyperthermic Intraperitoneal Chemotherapy in Ovarian Cancer. N Engl J Med. 2018;378(3):230–40. https://doi.org/10.1056/NEJMoa1708618
- Classe JM, Asselain B, Campion L, Berton D, Frenel JS, Lecuru F, et al. Survival Outcomes after Hyperthermic Intraperitoneal Chemotherapy for a First Ovarian Cancer Relapse: A Systematic Evidence-Based Review. Cancers (Basel). 2021;14(1). https://doi.org/10.3390/cancers14010172
- Aghajanian C, Goff B, Nycum LR, Wang YV, Husain A, Blank SV. Final overall survival and safety analysis of OCEANS, a phase 3 trial of chemotherapy with or without bevacizumab in patients with platinum-sensitive recurrent ovarian cancer. Gynecol Oncol. 2015;139(1):10–6. https://doi.org/10.1016/j.ygyno.2015.08.004
- Byrne AT, Ross L, Holash J, Nakanishi M, Hu L, Hofmann JI, et al. Vascular endothelial growth factor-trap decreases tumor burden, inhibits ascites, and causes dramatic vascular remodeling in an ovarian cancer model. Clin Cancer Res. 2003;9(15):5721–8.
- Mackey JR, Wood L, Nabholtz J, Jensen J, Venner P. A phase II trial of triamcinolone hexacetanide for symptomatic recurrent malignant ascites. J Pain Symptom Manage. 2000;19(3):193–9. https://doi.org/10.1016/S0885-3924(00)00106-8
- Heiss MM, Murawa P, Koralewski P, Kutarska E, Kolesnik OO, Ivanchenko VV, et al. The trifunctional antibody catumaxomab for the treatment of malignant ascites due to epithelial cancer: Results of a prospective randomized phase II/III trial. Int J Cancer. 2010;127(9):2209–21. https://doi.org/10.1002/ijc.25423
- Numnum TM, Rocconi RP, Whitworth J, Barnes MN. The use of bevacizumab to palliate symptomatic ascites in patients with refractory ovarian carcinoma. Gynecol Oncol. 2006;102(3):425–8. https://doi.org/10.1016/j.ygyno.2006.05.018
- Rossi L, Verrico M, Zaccarelli E, Papa A, Colonna M, Strudel M, et al. Bevacizumab in ovarian cancer: A critical review of phase III studies. Oncotarget. 2017;8(7):1238–405. https://doi.org/10.18632/oncotarget.13310
- Perren TJ, Swart AM, Pfisterer J, Ledermann JA, Pujade-Lauraine E, Kristensen G, et al. A phase 3 trial of bevacizumab in ovarian cancer. N Engl J Med. 2011;365(26):2484–96. https://doi.org/10.1056/NEJMoa1103799
- Burger RA, Brady MF, Bookman MA, Fleming GF, Monk BJ, Huang H, et al. Incorporation of bevacizumab in the primary treatment of ovarian cancer. N Engl J Med. 2011;365(26):2473–83. https://doi.org/10.1056/NEJMoa1104390
- Aghajanian C, Blank SV, Goff BA, Judson PL, Teneriello MG, Husain A, et al. OCEANS: a randomized, double-blind, placebo-controlled phase III trial of chemotherapy with or without bevacizumab in patients with platinum-sensitive recurrent epithelial ovarian, primary peritoneal, or fallopian tube cancer. J Clin Oncol. 2012;30(17):2039–45. https://doi.org/10.1200/JCO.2012.42.0505
- Pujade-Lauraine E, Hilpert F, Weber B, Reuss A, Poveda A, Kristensen G, et al. Bevacizumab combined with chemotherapy for platinum-resistant recurrent ovarian cancer: The AURELIA open-label randomized phase III trial. J Clin Oncol. 2014;32(13):1302–8. https://doi.org/10.1200/JCO.2013.51.4489
- Berek JS, Edwards RP, Parker LP, DeMars LR, Herzog TJ, Lentz SS, et al. Catumaxomab for the treatment of malignant ascites in patients with chemotherapy-refractory ovarian cancer: a phase II study. Int J Gynecol Cancer. 2014;24(9):1583–9. https://doi.org/10.1097/IGC.0000000000000286
- Gotlieb WH, Amant F, Advani S, Goswami C, Hirte H, Provencher D, et al. Intravenous aflibercept for treatment of recurrent symptomatic malignant ascites in patients with advanced ovarian cancer: a phase 2, randomised, double-blind, placebo-controlled study. Lancet Oncol. 2012;13(2):154–62. https://doi.org/10.1016/S1470-2045(11)70338-2
- Sjoquist KM, Espinoza D, Mileshkin L, Ananda S, Shannon C, Yip S, et al. REZOLVE (ANZGOG-1101): A phase 2 trial of intraperitoneal bevacizumab to treat symptomatic ascites in patients with chemotherapy-resistant, epithelial ovarian cancer. Gynecol Oncol. 2021;161(2):374–81. https://doi.org/10.1016/j.ygyno.2021.02.002
- Colombo N, Mangili G, Mammoliti S, Kalling M, Tholander B, Sternas L, et al. A phase II study of aflibercept in patients with advanced epithelial ovarian cancer and symptomatic malignant ascites. Gynecol Oncol. 2012;125(1):42–7. https://doi.org/10.1016/j.ygyno.2011.11.021
- Davies B, Brown PD, East N, Crimmin MJ, Balkwill FR. A synthetic matrix metalloproteinase inhibitor decreases tumor burden and prolongs survival of mice bearing human ovarian carcinoma xenografts. Cancer Res. 1993;53(9):2087–91.
- Watson SA, Morris TM, Parsons SL, Steele RJ, Brown PD. Therapeutic effect of the matrix metalloproteinase inhibitor, batimastat, in a human colorectal cancer ascites model. Br J Cancer. 1996;74(9):1354–8. https://doi.org/10.1038/bjc.1996.549
- Parsons SL, Watson SA, Steele RJ. Phase I/II trial of batimastat, a matrix metalloproteinase inhibitor, in patients with malignant ascites. Eur J Surg Oncol. 1997;23(6):526–31. https://doi.org/10.1016/S0748-7983(97)93077-8
- Beattie GJ, Smyth JF. Phase I study of intraperitoneal metalloproteinase inhibitor BB94 in patients with malignant ascites. Clin Cancer Res. 1998;4(8):1899–902.
- Zhao Y, Cao J, Melamed A, Worley M, Gockley A, Jones D, et al. Losartan treatment enhances chemotherapy efficacy and reduces ascites in ovarian cancer models by normalizing the tumor stroma. Proc Natl Acad Sci U S A. 2019;116(6):2210–9. https://doi.org/10.1073/pnas.1818357116
- Jatoi A, Nieva JJ, Qin R, Loprinzi CL, Wos EJ, Novotny PJ, et al. A pilot study of long-acting octreotide for symptomatic malignant ascites. Oncology. 2012;82(6):315–20. https://doi.org/10.1159/000337246
- Stuart GC, Nation JG, Snider DD, Thunberg P. Intraperitoneal interferon in the management of malignant ascites. Cancer. 1993;71(6):2027–30. https://doi.org/10.1002/1097-0142(19930315)71:6<2027::AID-CNCR2820710617>3.0.CO;2-C
- Rath U, Kaufmann M, Schmid H, Hofmann J, Wiedenmann B, Kist A, et al. Effect of intraperitoneal recombinant human tumour necrosis factor alpha on malignant ascites. Eur J Cancer. 1991;27(2):121–5. https://doi.org/10.1016/0277-5379(91)90467-R
- Katano M, Torisu M. New approach to management of malignant ascites with a streptococcal preparation, OK-432. II. Intraperitoneal inflammatory cell-mediated tumor cell destruction. Surgery. 1983;93(3):365–73.
- Torisu M, Katano M, Kimura Y, Itoh H, Takesue M. New approach to management of malignant ascites with a streptococcal preparation, OK-432. I. Improvement of host immunity and prolongation of survival. Surgery. 1983;93(3):357–64.
- Jandu N, Richardson M, Singh G, Hirte H, Hatton MW. Human ovarian cancer ascites fluid contains a mixture of incompletely degraded soluble products of fibrin that collectively possess an antiangiogenic property. Int J Gynecol Cancer. 2006;16(4):1536–44. https://doi.org/10.1111/j.1525-1438.2006.00624.x
- Rafehi S, Ramos Valdes Y, Bertrand M, McGee J, Prefontaine M, Sugimoto A, et al. TGFbeta signaling regulates epithelial-mesenchymal plasticity in ovarian cancer ascites-derived spheroids. Endocr Relat Cancer. 2016;23(3):147–59. https://doi.org/10.1530/ERC-15-0383
- Saini U, Naidu S, ElNaggar AC, Bid HK, Wallbillich JJ, Bixel K, et al. Elevated STAT3 expression in ovarian cancer ascites promotes invasion and metastasis: a potential therapeutic target. Oncogene. 2017;36(2):168–81. https://doi.org/10.1038/onc.2016.197
- Chen MW, Yang ST, Chien MH, Hua KT, Wu CJ, Hsiao SM, et al. The STAT3-miRNA-92-Wnt Signaling Pathway Regulates Spheroid Formation and Malignant Progression in Ovarian Cancer. Cancer Res. 2017;77(8):1955–67. https://doi.org/10.1158/0008-5472.CAN-16-1115
- Newsted D, Banerjee S, Watt K, Nersesian S, Truesdell P, Blazer LL, et al. Blockade of TGF-beta signaling with novel synthetic antibodies limits immune exclusion and improves chemotherapy response in metastatic ovarian cancer models. Oncoimmunology. 2019;8(2):e1539613. https://doi.org/10.1080/2162402X.2018.1539613
- Lee JM, Kohn EC. Proteomics as a guiding tool for more effective personalized therapy. Ann Oncol. 2010;21 Suppl 7:vii205-10. https://doi.org/10.1093/annonc/mdq375
- Davidson B, Espina V, Steinberg SM, Florenes VA, Liotta LA, Kristensen GB, et al. Proteomic analysis of malignant ovarian cancer effusions as a tool for biologic and prognostic profiling. Clin Cancer Res. 2006;12(3 Pt 1):791–9. https://doi.org/10.1158/1078-0432.CCR-05-2516
- Lee JM, Han JJ, Altwerger G, Kohn EC. Proteomics and biomarkers in clinical trials for drug development. J Proteomics. 2011;74(12):2632–41. https://doi.org/10.1016/j.jprot.2011.04.023
- Bi J, Newtson AM, Zhang Y, Devor EJ, Samuelson MI, Thiel KW, et al. Successful Patient-Derived Organoid Culture of Gynecologic Cancers for Disease Modeling and Drug Sensitivity Testing. Cancers (Basel). 2021;13(12). https://doi.org/10.3390/cancers13122901
- Beshiri ML, Tice CM, Tran C, Nguyen HM, Sowalsky AG, Agarwal S, et al. A PDX/Organoid Biobank of Advanced Prostate Cancers Captures Genomic and Phenotypic Heterogeneity for Disease Modeling and Therapeutic Screening. Clin Cancer Res. 2018;24(17):4332–45. https://doi.org/10.1158/1078-0432.CCR-18-0409
- Bartfeld S, Bayram T, van de Wetering M, Huch M, Begthel H, Kujala P, et al. In vitro expansion of human gastric epithelial stem cells and their responses to bacterial infection. Gastroenterology. 2015;148(1):126–36 e6. https://doi.org/10.1053/j.gastro.2014.09.042
- Huch M, Gehart H, van Boxtel R, Hamer K, Blokzijl F, Verstegen MM, et al. Long-term culture of genome-stable bipotent stem cells from adult human liver. Cell. 2015;160(1–2):299–312. https://doi.org/10.1016/j.cell.2014.11.050
- Sachs N, de Ligt J, Kopper O, Gogola E, Bounova G, Weeber F, et al. A Living Biobank of Breast Cancer Organoids Captures Disease Heterogeneity. Cell. 2018;172(1–2):373–86 e10. https://doi.org/10.1016/j.cell.2017.11.010
- Kopper O, de Witte CJ, Lohmussaar K, Valle-Inclan JE, Hami N, Kester L, et al. An organoid platform for ovarian cancer captures intra- and interpatient heterogeneity. Nat Med. 2019;25(5):838–49. https://doi.org/10.1038/s41591-019-0422-6
- Boretto M, Maenhoudt N, Luo X, Hennes A, Boeckx B, Bui B, et al. Patient-derived organoids from endometrial disease capture clinical heterogeneity and are amenable to drug screening. Nat Cell Biol. 2019;21(8):1041–51. https://doi.org/10.1038/s41556-019-0360-z
- Drost J, Clevers H. Organoids in cancer research. Nat Rev Cancer. 2018;18(7):407–18. https://doi.org/10.1038/s41568-018-0007-6
- Maru Y, Tanaka N, Itami M, Hippo Y. Efficient use of patient-derived organoids as a preclinical model for gynecologic tumors. Gynecol Oncol. 2019;154(1):189–98. https://doi.org/10.1016/j.ygyno.2019.05.005
- Hill SJ, Decker B, Roberts EA, Horowitz NS, Muto MG, Worley MJ, Jr., et al. Prediction of DNA Repair Inhibitor Response in Short-Term Patient-Derived Ovarian Cancer Organoids. Cancer Discov. 2018;8(11):1404–21. https://doi.org/10.1158/2159-8290.CD-18-0474
- Kim J, Koo BK, Knoblich JA. Human organoids: model systems for human biology and medicine. Nat Rev Mol Cell Biol. 2020;21(10):571–84. https://doi.org/10.1038/s41580-020-0259-3
- Velletri T, Villa CE, Cilli D, Barzaghi B, Lo Riso P, Lupia M, et al. Single cell-derived spheroids capture the self-renewing subpopulations of metastatic ovarian cancer. Cell Death Differ. 2021. https://doi.org/10.1038/s41418-021-00878-w
- Konecny G, Crohns C, Pegram M, Felber M, Lude S, Kurbacher C, et al. Correlation of drug response with the ATP tumorchemosensitivity assay in primary FIGO stage III ovarian cancer. Gynecol Oncol. 2000;77(2):258–63. https://doi.org/10.1006/gyno.2000.5728
- den Ouden JE, Zaman GJR, Dylus J, van Doornmalen AM, Mulder WR, Grobben Y, et al. Chemotherapy sensitivity testing on ovarian cancer cells isolated from malignant ascites. Oncotarget. 2020;11(49):4570–81. https://doi.org/10.18632/oncotarget.27827
- Matte I, Garde-Granger P, Bessette P, Piche A. Serum CA125 and ascites leptin level ratio predicts baseline clinical resistance to first-line platinum-based treatment and poor prognosis in patients with high grade serous ovarian cancer. Am J Cancer Res. 2019;9(1):160–70.
- Kerr EH, Frederick PJ, Egger ME, Stockard CR, Sellers J, DellaManna D, et al. Lung resistance-related protein (LRP) expression in malignant ascitic cells as a prognostic marker for advanced ovarian serous carcinoma. Ann Surg Oncol. 2013;20(9):3059–65. https://doi.org/10.1245/s10434-013-2878-9
- Brooks EA, Gencoglu MF, Corbett DC, Stevens KR, Peyton SR. An omentum-inspired 3D PEG hydrogel for identifying ECM-drivers of drug resistant ovarian cancer. APL Bioeng. 2019;3(2):026106. https://doi.org/10.1063/1.5091713
- Balasubramanian B, Venkatraman S, Myint KZ, Janvilisri T, Wongprasert K, Kumkate S, et al. Co-Clinical Trials: An Innovative Drug Development Platform for Cholangiocarcinoma. Pharmaceuticals (Basel). 2021;14(1). https://doi.org/10.3390/ph14010051
- Phan N, Hong JJ, Tofig B, Mapua M, Elashoff D, Moatamed NA, et al. A simple high-throughput approach identifies actionable drug sensitivities in patient-derived tumor organoids. Commun Biol. 2019;2:78. https://doi.org/10.1038/s42003-019-0305-x
- Pasch CA, Favreau PF, Yueh AE, Babiarz CP, Gillette AA, Sharick JT, et al. Patient-Derived Cancer Organoid Cultures to Predict Sensitivity to Chemotherapy and Radiation. Clin Cancer Res. 2019;25(17):5376–87. https://doi.org/10.1158/1078-0432.CCR-18-3590
- de Witte CJ, Espejo Valle-Inclan J, Hami N, Lohmussaar K, Kopper O, Vreuls CPH, et al. Patient-Derived Ovarian Cancer Organoids Mimic Clinical Response and Exhibit Heterogeneous Inter- and Intrapatient Drug Responses. Cell Rep. 2020;31(11):107762. https://doi.org/10.1016/j.celrep.2020.107762
- Chen H, Gotimer K, De Souza C, Tepper CG, Karnezis AN, Leiserowitz GS, et al. Short-term organoid culture for drug sensitivity testing of high-grade serous carcinoma. Gynecol Oncol. 2020;157(3):783–92. https://doi.org/10.1016/j.ygyno.2020.03.026
- Takahashi T. Organoids for Drug Discovery and Personalized Medicine. Annu Rev Pharmacol Toxicol. 2019;59:447–62. https://doi.org/10.1146/annurev-pharmtox-010818-021108