17
Maximizing Local Access to Therapeutic Deliveries in Glioblastoma. Part I: Targeted Cytotoxic Therapy
Waldemar Debinski1 • Waldemar Priebe2 • Stephen B. Tatter1,3
1Brain Tumor Center of Excellence, Wake Forest Baptist Medical Center Comprehensive Cancer Center, Winston Salem, NC, USA; 2Department of Experimental Therapeutics, Division of Cancer Medicine, University of Texas MD Anderson Cancer Center, Houston, TX, USA; 3Deparment of Neurosurgery, Wake Forest Baptist Medical Center, Winston Salem, NC, USA
Abstract: Glioblastoma (GBM), a primary brain tumor, remains an unmet medical need. One of the major obstacles to GBM treatment is the adequate properties of drugs. Complex pathobiology of GBM, including local invasion and intratumoral heterogeneity, represent major challenges to generating effective therapies. We discuss here the design of targeted cytotoxic drugs with an increased access to tumors and pathophysiologically important tumor compartments. Our research and others’ have shown that interleukin 13 receptor alpha 2 (IL-13RA2), EphA2, and EphA3 receptors are overexpressed in most patients with GBM, but not in normal brain, and also in spontaneous canine high-grade gliomas like GBM, an excellent translational model of GBM. These receptors and also the EphB2 receptor are overexpressed and are functional in several GBM compartments involved in tumor progression and/or resistance to therapies. We pursue the novel idea of targeting all four receptors with one targeted cytotoxic compound (QUAD-CTX). We are constructing a molecularly targeted anti-GBM drug that (i) may not require patient prescreening, (ii) will attack most tumor compartments known to be pathobiologically important, and (iii) performs these functions in one pharmaceutical entity, so it will be suitable for monotherapy. We thus wish to take advantage of a unique opportunity to produce an off-the-shelf, highly specific, molecularly targeted drug candidate suitable to treat perhaps even all patients with GBM. We envision that this “molecular resection” will translate into clear-cut durable responses in patients suffering from this dreadful disease.
Keywords: Convection-enhanced delivery; Glioblastoma; IL-13RA2; Receptors; Targeted cytotoxins
Author for correspondence: Waldemar Debinski, Brain Tumor Center of Excellence, Wake Forest Baptist Medical Center, NRC/Commons Rm 210A, 1 Medical Center Boulevard, Winston Salem, NC 27157, USA. E-mail: debinski@wakehealth.edu
Doi: http://dx.doi.org/10.15586/codon.glioblastoma.2017.ch17
In: Glioblastoma. Steven De Vleeschouwer (Editor), Codon Publications, Brisbane, Australia ISBN: 978-0-9944381-2-6; Doi: http://dx.doi.org/10.15586/codon.glioblastoma.2017
Copyright: The Authors.
Licence: This open access article is licenced under Creative Commons Attribution-NonCommercial 4.0 International (CC BY 4.0). https://creativecommons.org/licenses/by-nc/4.0/
Introduction
Effective therapy of glioblastoma (GBM) remains an elusive goal. Despite nearly 80 years of effort, only 1 month per decade has been added to the mean survival rate of GBM patients, and the 2-year survival rate remains below 25% with practically no cures (1). Recently, several highly anticipated efficacy trials including antiangiogenic therapies all failed in patients with GBM (2–5). Similarly, inhibiting a vital signaling pathway in a single compartment of GBM, namely, glioma stem-like cells (GSCs), conferred no clinical benefit (6, 7). Many small-molecule inhibitors have not progressed beyond early-phase trials based on little objective benefit (8, 9). On the other hand, immunotherapy trials showed promising results, including dendritic cell vaccination against IL-13RA2 (10), among other targets, and peptide vaccination against EGFRvIII (11). Although the vaccination against EGFRvIII in recently finished efficacy trial reproduced results from Phase I and II, the control group unexpectedly showed an increase in overall survival by 40% from previously observed (12). This is reminiscent of a similar happening when an IL-13-based cytotoxin was used in Phase III PRECISE trial (13, 14). Of interest, a medical device called Optune (Novocure) generating electric fields demonstrated clinical efficacy (15, 16). In short, GBM remains refractory to standard and experimental treatments. Predictions about translational potential of virtually all therapeutic approaches have not been realized thus far.
High mortality in GBM is often attributed to its complex pathobiology, including high cellularity, neovascularization, hypoxia/necrosis, immune cell infiltration, and local invasion (17). Moreover, GSCs may play an important role in GBM progression/recurrence and resistance to therapies like chemotherapy or radiation (18, 19). Recently, four genomic subtypes of GBM were delineated: proneural, neural, mesenchymal, and classical (20, 21), supportive of the complex pathobiological nature of GBM. Common treatment approaches involve surgery (22), radiation therapy (23), and various chemotherapeutic regimens (24, 25).
Other major obstacles to GBM treatment is the presence of barriers like blood– brain barrier (BBB) and blood–brain tumor barrier (BBTB), limiting or outrightly preventing any diffusion of drugs into tumors when given systemically (Figure 1). We believe that we can improve treatment of GBM by addressing crucial issues in drug design and their delivery by maximizing drugs access to tumors and their targets. This can be achieved by generating anti-GBM drugs that attack concomitantly multiple GBM compartments that are responsible for tumor progression and resistance to the existing therapies and experimental therapies. For example, we can aim at four molecular targets like IL-13RA2, EphA2, EphA3, and EphB2 receptors that are specifically overexpressed on GBM tumor cells.
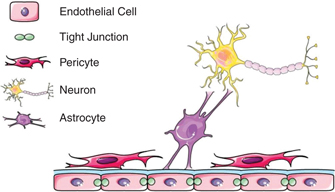
Figure 1 Schematic drawing of the blood-brain barrier. The blood–brain barrier is comprised of neurovascular units. Endothelial cells are connected by tight junctions and share a basement lamina with pericytes. Astrocytic end-feet are also at the basement lamina interface and these cells interact with neurons (This figure was developed using Servier Medical Art (http://www.servier.com/Powerpointimage-bank) under a Creative Commons attribution 3.0 Unported License). (Adapted from Pharmaceutics 2015;7(3):175–187.)
Targeted Cytotoxic Therapy of GBM
GBM is the most common primary brain tumor in adults, and the median survival is only ~14.5 months (1, 26). We discovered that interleukin 13 receptor alpha 2 (IL-13RA2) and EphA2 receptor are overexpressed in most patients with GBM, but not in normal brain (27–31), and also in spontaneous canine GBM, an excellent translational model of GBM (32–35). Expression of IL-13RA2 and EphA2 is partially overlapping; hence, the combined overexpression is ~90% in patients with GBM (31). IL-13RA2 and EphA2 are targets for multiple therapeutic approaches currently in the clinic or under preclinical evaluation (36–52). The first generation of an IL-13-based cytotoxin produced in our laboratory, which nonspecifically targeted IL-13RA2, demonstrated clinical efficacy in patients with recurrent GBM (13, 53–55). We developed a protocol for a Phase I clinical trial in dogs with gliomas (see also chapter 21, page 405) and began the trial using a cocktail of cytotoxins targeting IL-13RA2 (using a variant of IL-13 as a specific targeting ligand) and EphA2 receptor (based on ephrin A1, a ligand for the EphA2 receptor). The drugs are given locoregionally through convection-enhanced delivery (CED) using anti-reflux catheters (Figure 2 in Chapter 21). We have already seen significant antitumor responses in this dose-finding trial.
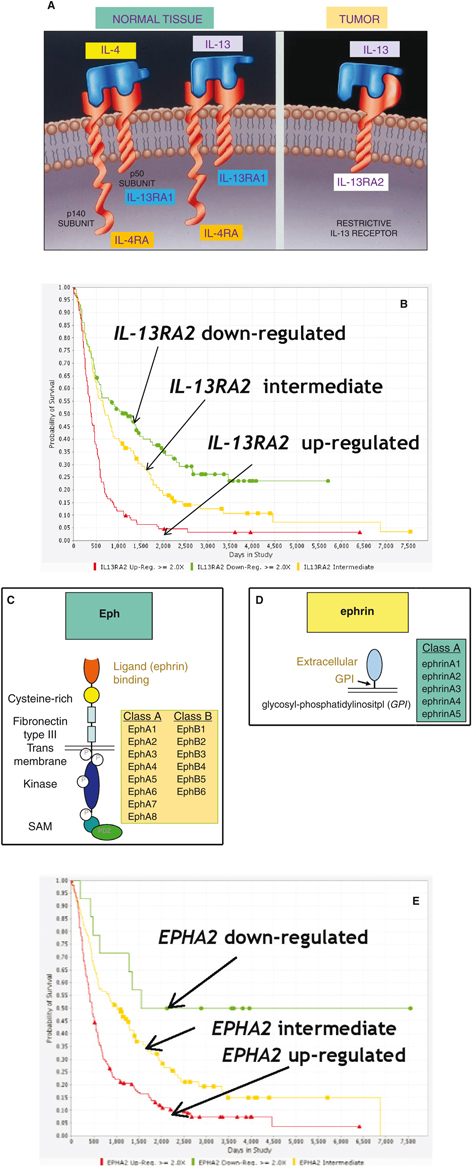
Figure 2 IL-13RA2 and EphA2 in cancer. (A) Schemata of normal tissue, IL-13RA1/IL-4A, and tumor-associated receptor, IL-13RA2 for IL-13 (adapted from Sci Med 1998;5:36–42). (B) Kaplan–Meier survival plots with differential IL-13RA2 gene expression. REMBRANDT database of human gliomas was used for calculations (https://caintegrator.nci.nih.gov/rembrandt/). All differences were statistically significant. (C, D) Schemata of Eph receptors and their ligands, ephrinAs, respectively. (E) Kaplan–Meier survival plots with differential EphA2 gene expression. REMBRANDT database of human gliomas was used for calculations as in B. All differences were statistically significant.
Our research and others’ have shown that IL-13RA2, EphA2, and also EphA3 (Figure 2) are widely present in various compartments of GBM tumors. For example, all three receptors are expressed in tumor cells of the core of GBM tumors. Importantly, IL-13RA2, EphA2, and EphA3 are present on tumor-infiltrating cells, while EphA2 is also overexpressed in tumor neovasculature (56, 57). Interestingly, IL-13RA2, EphA2, and EphA3 were associated with, and play crucial roles in, the pathobiology of GSCs. IL-13RA2 is abundant in cells isolated as GSCs from GBM (58–60) and contributes to their cell stem properties (61). EphA2 and EphA3 drive self-renewal and tumorigenicity of GSCs (62–64). Finally, the EphA3 receptor can be readily detected in GBM-infiltrating cells of monocytic origin, tumor-associated macrophages (TAM) (Figure 3). Thus, collectively, IL-13RA2, EphA2, and EphA3 are expressed in several GBM compartments documented to be involved in tumor progression and/or resistance to therapies (18, 19). Of importance, ephrin-A5 (eA5) binds EphA2 and EpA3 receptors and also the EphB2 (17, 65, 66) receptor, all present in abundance in GBM tumors, but not in normal brain. Here, we discuss the novel idea of targeting all four receptors with one pharmaceutical compound. We are exploiting the favorable properties of our previously generated IL-13 variants and those of engineered eA5, to construct a human IgG1 scaffold-based single pharmaceutical compound. The multivalent compound will bind IL-13RA2, EphA2, EphA3, and EphB2 and deliver a catalyst(s) to GBM tumors, specifically killing tumor cells and abnormal cells of the tumor environment. Such an approach offers a unique opportunity to gain an increased access to tumor compartments of high resistance, or poor availability, to current treatment modalities.
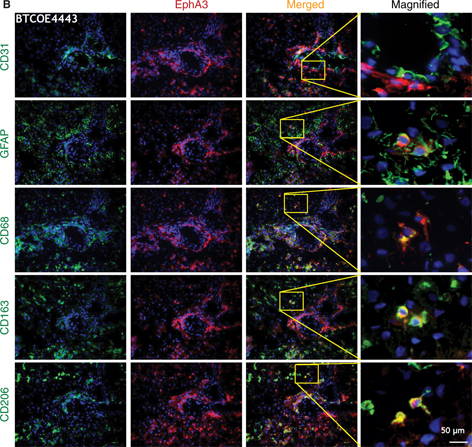
Figure 3 EphA3 receptor in GBM. Immunofluorescent staining of EphA3 (red) and CD31, GFAP, CD68, CD163 and CD206 (green) on consecutive sections of the same GBM specimen. Nuclei were stained with DAPI (blue). (Adapted from Oncotarget 2016;7(37):59860–59876.)
ATTRACTIVE MOLECULAR TARGETS IN GBM
We discovered the first receptor target overexpressed in most GBM patients, but not in normal brain: IL-13RA2 (29). IL-13RA2 is a monomeric receptor to which only IL-13 binds, unlike its normal tissue counterpart, IL-13RA1/IL-4A, which binds IL-13 and IL-4 (5) (Figure 2A). IL-13RA2 is (i) associated with GBM patients’ survival, (ii) expressed preferentially in a GBM mesenchymal subtype, and (iii) its gene, based on TCGA data, is overexpressed in 58% of patients (58; Figure 2B) and in the protein in up to 75% of GBM cases (35). IL-13RA2 was readily detected in cells isolated as GSC (59, 60) and appears to contribute to GBM cell stemness. For example, GBM cells selected for lack of IL-13RA2 have a significantly lower stem cell–like forming and tumorigenic potential (61). This observation provides strong rationale for treatments eliminating IL-13RA2 positive cells. IL-13RA2 may influence intracellular signaling (67) and may be a signaling molecule (68).
Our continuous efforts to find pharmaceutically tractable molecular targets led to discovery of the EphA2 receptor in GBM (31, 69–71). EphA2 belongs to the largest protein tyrosine kinase receptor family in eukaryotes (72–75) (Figure 2C); these receptors are bound by natural ligands called ephrins (Figure 2D). EphA2 is over-expressed in ~ 60% of patients with GBM (31, 76), but jointly with IL-13RA2 it is over-expressed in ~90% of all GBM, while absent in normal brain (31). Expression of EphA2 and EphA2 correlates with glioma patients’ survival (75) (see also Figure 2E) (77, 78). IL-13RA2 and EphA2 exist in a significant proportion of locally infiltrating GBM cells, and EphA2 is overexpressed on abnormal endothelium of tumor-associated vessels (52, 79). EphA2 activation by its preferred ligand, ephrin-A1 (eA1), induces prominent, dose-dependent inhibitory effects on anchorage-independent growth and invasiveness of GBM cells (79, 80). The EphA2/eA1 system function in GBM is complex; the receptor is oncogenic when ligand unactivated, but tumor suppressing when activated by eA1 (68, 69). EphA2 is also important for the self-renewing and tumorigenic potential of GSCs (62, 63). Thus, IL-13RA2 and EphA2 are attractive molecular targets for urgently needed targeted combinatorial therapy (31, 80, 81).
Most recently, we found that another receptor of the EphA subfamily, the EphA3 receptor, is overexpressed in GBM (Figure 3). The gene for EphA3 was highly upregulated in G48a GBM cell tumorspheres (56) and even more so in nonpassaged GBM cells. Others found the EphA3 receptor is important for the self-renewing potential and tumorigenicity of GSCs (64). Interestingly, the distribution of EphA2 and EphA3 receptors only partially overlaps. For example, EphA3 receptors are found in cells of monocytic origin infiltrating GBM (82) like TAMs (Figure 3). The difference in these receptors’ distribution also agrees with the existence of multiple phenotypic types of GSCs (83). EphA3 often co-localized with a GSC marker Nestin in situ, in accordance with a recent report (64). EphA3 did not co-localize with the endothelial cell marker CD31 (Figure 3). Microglia/macrophages variably infiltrate gliomas and contribute to the total tumor mass (82). Three markers of monocyte/macrophage lineage (CD68, CD163, and CD206) co-stained with EphA3 on a subpopulation of cells within the tumor and surrounding the tumor neovasculature (64). This novel finding widens the spectrum of GBM compartments that can be exploited as targets in molecular anti-GBM therapies using Eph receptors.
PROMISING TREATMENT BYPASSING THE BBB
We and others have continued to optimize CED as a minimally invasive approach (33–35, 84–97). This way of drugs delivery is discussed in Part II of the chapter (page 347). A critical therapeutic advantage in using cytotoxins via CED is that they cause death of targeted cells (70). Even though the GBM tumor environment is highly immunosuppressive (98, 99) and patients with GBM are immunosuppressed (100, 101), a large number of killed tumor cells provides a “danger signal” and evokes effective antitumor immune responses. Our published (41) and unpublished observations suggested, and another study demonstrated directly (102), the existence of effective immune responses in preclinical studies with cytotoxic proteins. Importantly, the “in situ vaccine” effect of a treatment causing cell death is also the principal mechanism of antitumor action when using oncolytic viruses delivered directly to tumors using CED (6, 103). In this approach, the virus is delivered only to a portion of GBM tumors through a single catheter, but cell death at the site and near the virus injection appears sufficient to produce readily measurable immune responses in patients and subsequent antitumor effects (6, 103). Therefore, if one cannot distribute cytotoxins perfectly throughout the whole tumor and its vicinity in all patients at all times, the death of most cells in tumors during each treatment will result in an antitumor vaccination effect meaning new influx of immune cells responsible for the whole mechanism of response. This principle is tested in other cancers like melanoma; the viral gene therapy drug, Imlygic, has been approved by the FDA (104). Conceivably, the addition of immune checkpoint inhibitors should result in potentiation of such responses (105, 106).
INCREASING DRUGS ACCESS TO TUMOR COMPARTMENTS AND OPTIMIZING CED FOR EFFECTIVE GBM TREATMENT
The clinical results obtained with the first generation of IL-13-based cytotoxin, huIL-13-PE38QQR, which is a fusion protein between a wild type IL-13 and a modified pseudomonas exotoxin A (PE) represent promising translational starting point to improve treatment of patients with GBM. Early-phase trials with huIL-13-PE38QQR showed up to 56 weeks of median survival and a number of long-lasting responses (107). Importantly, the efficacy trial extended the lives of patients with recurrent GBM by almost 50%, but the control arm had extended from previously observed survivals and the favorable difference did not achieve statistical significance (13). The ways to improve the CED are described in further detail in chapter 18 (page 359) and chapter 21 (Page 405).
TARGETING EPH RECEPTORS SIMULTANEOUSLY
As pointed out above, IL-13RA2, EphA2, EphA3, and EphB2 are overexpressed, functionally important, and linked to survival in patients with GBM. They are all pharmaceutically targetable as demonstrated in preclinical and clinical studies. These receptors are distributed among several tumor compartments important for progression of GBM. Debinski’s group has long been advocating attacking GBM with a combinatorial approach (31, 80, 81). Given that only two modified ligands may be needed to generate a drug delivery system targeting all four receptors, we have a completely new opportunity for combinatorial therapy and highly increased drug access in a complex disease of dismal prognosis, with just one pharmaceutical agent.
eA5 can bind and induce internalization of the Eph receptors A2, A3, and EphB2. Hence, we produced a dimeric form of eA5 in a fusion with an Fc fragment of human IgG1, eA5-Fc (Figure 4A) (56). We also made an eA5-Fc-PE38QQR cytotoxin chemical conjugate (Figure 4A). eA5-Fc-PE38QQR killed U-251 MG, U-373 MG, and G48a GBM cells very efficiently and specifically (Figure 4B). The IC50 of eA5-Fc-PE38QQR was in the range of 10−11 M. To confirm the specificity of the cytotoxin in targeting EphA2 and EphA3, the three GBM cell lines were pretreated with either eA1-Fc or eA5-Fc at 10 μg/mL for 1 h. As expected, the cytotoxin was less active on the three cell lines tested when pretreated with eA1-Fc, which binds only the EphA2 receptor, and completely lost its activity when cells were pretreated with eA5-Fc, which binds EphA2, EphA3, and EphB2 (Figure 4B). Even though the readings in colorimetric cell viability assay were not reaching 100% kill, the live/dead assay (Life Technologies) demonstrated that vast majority of GBM cells were dead at 10 ng/ml of conjugate concentration and almost all were dead at 100 ng/ml of conjugate (56).
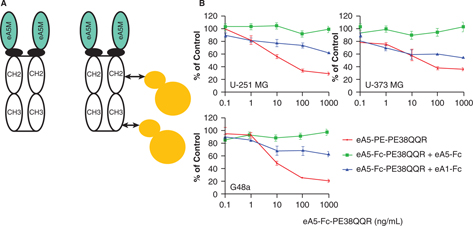
Figure 4 EA5-Fc-PE38QQR kills GBM tumor cells, specifically targeting both EphA3 and EphA2 receptors. (A) The structure of an eA5-Fc and eA5-Fc chemically conjugated to PE38QQR (right). Opposite arrows represent chemical conjugation. Closed small ovals represent hinge regions; thin straight lines represent disulfide bonds. Orange circles are the domains of PE: smaller = D2 and larger = Domain III. (B) Cell viability assay on GBM cell lines treated with eA5-Fc-PE38QQR for 48 h or pretreated with either eA1-Fc or eA5-Fc. (Adapted from Oncotarget 2016;7(37):59860–59876.)
TARGETING EPH RECEPTORS AND IL-13RA2 SIMULTANEOUSLY
eA5-Fc interacts with EphA2, EphA3, and EphB2 receptors. To further widen the reach of a targeting agent, we are incorporating mutated IL-13 (IL-13M), which has dramatically altered reactivity toward the normal tissue receptor, IL-13RA1/IL-4RA, but not toward the tumor-associated receptor, IL-13RA2 (41, 108–111) into the eA5-Fc construct (Figures 2A and 5A). The very first construct retained an ability to bind to the EphA2 receptor and IL-13RA2. Next, we will produce a chemical conjugate between eA5M-Fc-IL-13M and PE38QQR to demonstrate feasibility of the QUAD-CTX approach in a direct way similarly to eA5-Fc-PE38QQR. We will also make conjugates of eA5-Fc-IL-13M with chemotherapeutics like WP936 (112). This will eliminate several potential problems related to possible systemic delivery of toxin-based therapeutics.
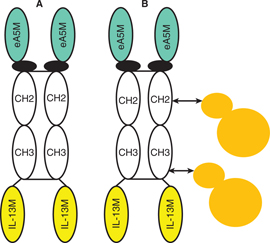
Figure 5 Design of a QUAD-CTX. (A) Schemata of eA5M-Fc-IL-13M in which the moieties of eA5M and IL-13M are targeting ligands. (B) Schemata of eA5M-Fc-IL-13M conjugated to PE38QQR. Closed small ovals represent hinge regions. Opposite arrows represent chemical conjugation. Closed small blue ovals represent chemotherapeutic. Filled triangles represent chemotherapeutic. Orange circles are the domains of PE: smaller = D2, and larger = Domain III.
QUAD-CTX BASED ON SCFV RECEPTOR TARGETING
We are generating another type of QUAD-CTX drug candidate in which we will use single-chain (sc) Fv fragments of antibodies (scFv) directed individually against the three Eph receptors: A2, A3, and B2. It will be also based on a human IgG1 scaffold similarly to eA5M-Fc-IL-13M. We have already made the first step in generating a quadrivalent scFv(EphA2)-scFv(EphA3)-scFv(EphB2)-Fc-IL-13M. We have produced a bivalent scFv(EphA2)-Fc-IL-13M (Figure 6A). We will stepwise introduce scFvs for EphA3 and EphB2 receptors (Figure 6B) with various placement configuration and test for the exhibition of expected binding properties. Once it is made, the quadrivalent ligand will be conjugated to either modified toxins or a chemotherapeutic like WP936 (Figure 6C).
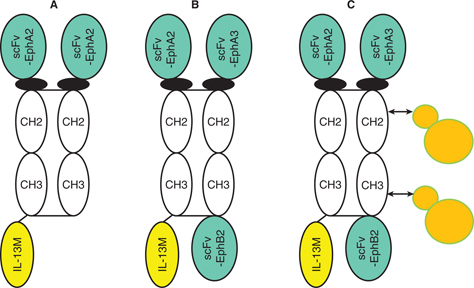
Figure 6 Construction of a QUAD-CTX based on scFvs. (A) Bivalent scFv(EphA2)-Fc-IL-13M construct. (B) Schemata of a quadrivalent scFv(EphA2)-scFv(EphA3)-scFv(EphB2)-Fc-IL-13M construct. (C) Schemata of a quadrivalent scFv(EphA2)-scFv(EphA3)-scFv(EphB2)-Fc-IL-13M chemical conjugate with toxic load. Closed small ovals represent hinge regions; thin straight lines represent disulfide bonds. Opposite arrows represent chemical conjugation. Closed small blue ovals represent either toxins or chemotherapeutic.
Conclusion
We have discussed our idea to target the three molecular targets that we identified in GBM: IL-13RA2, EphA2, and EphA3, and yet another receptor, EphB2 that are specifically overexpressed on GBM tumor cells as well. We are developing a cytotoxic drug of highly unique properties that can recognize four receptors, QUAD-CTX. Also, because virtually all GBM patients have these receptors in abundance, prescreening patients for this treatment may not be necessary. Thus, our new design will increase drug access to hard-to-target GBM compartments believed to be responsible for dismal prognosis of the disease. Thus, despite significant obstacles in drug delivery to GBM and the high complexity and heterogeneity of GBM, an off-the-shelf drug used as monotherapy can represent an effective combinatorial therapy approach using both passive and active immunotherapy.
Acknowledgment: This work was supported by NCI R01 CA74145, R01 CA139099, P01 CA 207206 and Thomas K. Hearn, Pratto, Dallas Ray Swing, and JS Farmer III Family Funds for Brain Tumor Research.
Conflict of Interest: Dr. Waldemar Debinski is an inventor on the patent applications filed or patents issued on the subject of this chapter. He is also a Scientific Advisor to Targepeutics, Inc. and holds equity in the company.
Copyright and permission statement: To the best of our knowledge, the materials included in this chapter do not violate copyright laws. All original sources have been appropriately acknowledged and/or referenced. Where relevant, appropriate permissions have been obtained from the original copyright holder(s).
REFERENCES
- Stupp R, Mason WP, van den Bent MJ, Weller M, Fisher B, Taphoorn MJ, et al. Radiotherapy plus concomitant and adjuvant temozolomide for glioblastoma. N Engl J Med. 2005;352:987–96. http://dx.doi.org/10.1056/NEJMoa043330
- Friedman HS, Prados MD, Wen PY, Mikkelsen T, Schiff D, Abrey LE, et al. Bevacizumab alone and in combination with irinotecan in recurrent glioblastoma. J Clin Oncol. 2009;27:4733–40. http://dx.doi.org/10.1200/JCO.2008.19.8721
- Gilbert MR, Dignam JJ, Armstrong TS, Wefel JS, Blumenthal DT, Vogelbaum MA, et al. A randomized trial of bevacizumab for newly diagnosed glioblastoma. N Engl J Med. 2014 Feb 20;370(8):699–708. http://dx.doi.org/10.1056/NEJMoa1308573
- Chinot OL, Wick W, Mason W, Henriksson R, Saran F, Nishikawa R, et al. Bevacizumab plus radiotherapy-temozolomide for newly diagnosed glioblastoma. N Engl J Med. 2014 Feb 20;370(8):709–22. http://dx.doi.org/10.1056/NEJMoa1308345
- Stupp R, Hegi ME, Gorlia T, Erridge SC, Perry J, Hong YK, et al. Cilengitide combined with standard treatment for patients with newly diagnosed glioblastoma with methylated MGMT promoter (CENTRIC EORTC 26071-22072 study): A multicentre, randomised, open-label, phase 3 trial. Lancet Oncol. 2014 Sep;15(10):1100–8. http://dx.doi.org/10.1016/S1470-2045(14)70379-1
- Desjardins A, Vlahovic G, Friedman HS. Vaccine therapy, oncolytic viruses, and gliomas. Oncology (Williston Park). 2016 Mar;30(3):211–18.
- Sloan AE, Nock CJ, Kerstetter A, Supko J, Ye X, Barnholtz-Sloan JS, et al. Targeting glioma stem cells (GSC): A biomarker and Phase II study of GDC-0449 in patients with recurrent glioblastoma multi-forme. Neuro Oncol. 2012 Oct;14(Suppl 6):vi101–vi105.
- Wick W, Weller M, Weiler M, Batchelor T, Yung AW, Platten M. Pathway inhibition: Emerging molecular targets for treating glioblastoma. Neuro Oncol. 2011 Jun;13(6):566–79. http://dx.doi.org/10.1093/neuonc/nor039
- Nathanson DA, Gini B, Mottahedeh J, Visnyei K, Koga T, Gomez G, et al. Targeted therapy resistance mediated by dynamic regulation of extrachromosomal mutant EGFR DNA. Science. 2014; 343(6166):72–6. http://dx.doi.org/10.1126/science.1241328
- ImmunoCellular therapeutics phase II study demonstrates that glioblastoma patients live longer without disease progression when treated with ICT-107. http://investors.imuc.com/releasedetail.cfm?ReleaseID=813442.
- Reardon D, Schuster J, Tran D, Fink K, Nabors L, Li G, et al. IT-30. ReACT: A phase II study of rindopepimut vaccine (CDX-110) plus bevacizumab in relapsed glioblastoma. Neuro Oncol. 2014;16(Suppl 5):v116.
- Malkki H. Glioblastoma vaccine therapy disappointment in Phase III trial. Nature Rev Neurol. 2016;12:190. http://dx.doi.org/10.1038/nrneurol.2016.38
- Sampson JH, Archer G, Pedain C, Wembacher-Schröder E, Westphal M, Kunwar S, et al. Poor drug distribution as a possible explanation for the results of the PRECISE trial. J Neurosurgery. 2010;113:301–309. http://dx.doi.org/10.3171/2009.11.JNS091052
- Kunwar S, Chang S, Westphal M, Vogelbaum M, Sampson J, Barnett G, et al. Phase III randomized trial of CED of IL13-PE38QQR vs Gliadel wafers for recurrent glioblastoma. PRECISE Study Group. Neuro Oncol. 2010 Aug;12(8):871–881. http://dx.doi.org/10.1093/neuonc/nop054
- Stupp R, Wong ET, Kanner AA, Steinberg D, Engelhard H, Heidecke V, et al. NovoTTF-100A versus physician’s choice chemotherapy in recurrent glioblastoma: A randomised phase III trial of a novel treatment modality. Eur J Cancer. 2012;48(14):2192–202. http://dx.doi.org/10.1016/j.ejca.2012.04.011
- Stupp R, Taillibert S, Kanner AA, Kesari S, Steinberg DM, Toms SA, et al. Maintenance therapy with tumor-treating fields plus temozolomide vs temozolomide alone for glioblastoma: A randomized clinical trial. JAMA. 2015 Dec 15;314(23):2535–43. http://dx.doi.org/10.1001/jama.2015.16669
- Vartanian A, Singh SK, Agnihotri S, Jalali S, Burrell K, Aldape KD, et al. GBM’s multifaceted landscape: highlighting regional and microenvironmental heterogeneity. Neuro Oncol. 2014 Aug;16(8):1025–6. http://dx.doi.org/10.1093/neuonc/nou121
- McLendon RE, Rich JN. Glioblastoma stem cells: A neuropathologist’s view. J Oncol. 2011;2011:397195. http://dx.doi.org/10.1155/2011/397195
- Singh SK, Hawkins C, Clarke ID, Squire JA, Bayani J, Hide T, et al. Identification of human brain tumor initiating cells. Nature. 2004;432(7015):396–401. http://dx.doi.org/10.1038/nature03128
- Verhaak RG, Hoadley KA, Purdom E, Wang V, Qi Y, Wilkerson MD, et al. Cancer Genome Atlas Research Network. Integrated genomic analysis identifies clinically relevant subtypes of glioblastoma characterized by abnormalities in PDGFRA, IDH1, EGFR, and NF1. Cancer Cell. 2010 Jan 19;17(1):98–110. http://dx.doi.org/10.1016/j.ccr.2009.12.020
- Brennan CW, Verhaak RG, McKenna A, Campos B, Noushmehr H, Salama SR, et al. TCGA Research Network. The somatic genomic landscape of glioblastoma. Cell. 2013 Oct 10;155(2):462–77. http://dx.doi.org/10.1016/j.cell.2013.09.034
- Sanai N, Berger MS. Recent surgical management of gliomas. Adv Exp Med Biol. 2012;746:12–25. http://dx.doi.org/10.1007/978-1-4614-3146-6_2
- Chan MD, Tatter SB, Lesser G, Shaw EG. Radiation oncology in brain tumors: Current approaches and clinical trials in progress. Neuroimaging Clin N Am. 2010;20(3):401–8. http://dx.doi.org/10.1016/j.nic.2010.04.005
- Blakeley J, Grossman SA. Chemotherapy with cytotoxic and cytostatic agents in brain cancer. Handb Clin Neurol. 2012;104:229–54. http://dx.doi.org/10.1016/B978-0-444-52138-5.00017-7
- Reardon DA, Perry JR, Brandes AA, Jalali R, Wick W. Advances in malignant glioma drug discovery. Expert Opin Drug Discov. 2011 Jul;6(7):739–53. http://dx.doi.org/10.1517/17460441.2011.584530
- Louis DN, Perry A, Reifenberger G, von Deimling A, Figarella-Branger D, Cavenee WK, et al. The 2016 World Health Organization classification of tumors of the central nervous system: A summary. Acta Neuropathol. 2016 Jun;131(6):803–20. http://dx.doi.org/10.1007/s00401-016-1545-1
- Debinski W, Gibo DM, Hulet SW, Connor JR, Gillespie GY. Receptor for interleukin 13 is a marker and therapeutic target for human high grade gliomas. Clin Cancer Res. 1999;5:985–90.
- Mintz A, Gibo DM, Webb (Slagle) B, Christensen AD, Debinski W. IL13Rα2 is a glioma-restricted receptor for IL13. Neoplasia. 2002;4:388–99. http://dx.doi.org/10.1038/sj.neo.7900234
- Debinski W. An immune regulatory cytokine receptor and glioblastoma multiforme: An unexpected link. Crit Rev Oncog. 1998;9:255–68.
- Debinski W, Gibo DM. Molecular expression analysis of restrictive receptor for interleukin 13, a brain tumor-associated cancer/testis antigen. Mol Med. 2000;6:440–9.
- Wykosky J, Gibo DM, Stanton C, Debinski W. IL-13 Receptor alpha-2, EphA2, and Fra-1 as molecular denominators of high-grade astrocytomas and specific targets for combinatorial therapy. Clin Cancer Res. 2008;14:199–208. http://dx.doi.org/10.1158/1078-0432.CCR-07-1990
- Debinski W, Dickinson P, Rossmeisl JH, Robertson J, Gibo DM. New agents for targeting of IL-13RA2 expressed in primary human and canine brain tumors. PLoS One. 2013 Oct 16;8(10):e77719. http://dx.doi.org/10.1371/journal.pone.0077719
- Candolfi M, Curtin JF, Nichols WS, Muhammad AG, King GD, Pluhar GE, et al. Intracranial glioblastoma models in preclinical neuro-oncology: Neuropathological characterization and tumor progression. J Neurooncol. 2007 Nov;85(2):133–48. http://dx.doi.org/10.1007/s11060-007-9400-9
- Dickinson PJ, LeCouteur RA, Higgins RJ, Bringas JR, Larson RF, Yamashita Y, et al. Canine spontaneous glioma: A translational model system for convection-enhanced delivery. Neuro Oncol. 2010;12:928–40. http://dx.doi.org/10.1093/neuonc/noq046
- Dickinson PJ, LeCouteur RA, Higgins RJ, Bringas JR, Roberts B, Larson RF, et al. Canine model of convection-enhanced delivery of liposomes containing CPT-11 monitored with real-time magnetic resonance imaging: Laboratory investigation. J Neurosurgery. 2008;108:989–98. http://dx.doi.org/10.3171/JNS/2008/108/5/0989
- Okano F, Storkus WJ, Chambers WH, Pollack IF, Okada H. Identification of a novel HLA-A*0201restricted, cytotoxic T lymphocyte epitope in a human glioma-associated antigen, interleukin 13 receptor alpha 2 chain. Clin Cancer Res. 2002;8:2851–5.
- Mintz A, Gibo DM, Madhankumar AB, Cladel NM, Christensen ND, Debinski W. Protein and DNA-based active immunotherapy targeting interleukin 13 receptor alpha 2. Cancer Biother Radiopharm. 2008;23:581–9. http://dx.doi.org/10.1089/cbr.2008.0462
- Zhou G, Ye G-J, Debinski W, Roizman B. Genetic engineering of a herpes virus 1 vector dependent on the IL-13R-2 receptor for entry into cells: Interaction of glycoprotein D with its receptors is independent of the fusion of the envelope and the plasma membrane. Proc Natl Acad Sci. 2002;99: 15124–15129. http://dx.doi.org/10.1073/pnas.232588699
- Kahlon KS, Brown C, Cooper LJN, Raubitschek A, Forman SJ, Jensen MC. Specific recognition and killing of glioblastoma multiforme by interleukin 13-zetakine redirected cytolytic T cells. Cancer Res. 2004;64:9160–7. http://dx.doi.org/10.1158/0008-5472.CAN-04-0454
- Chunbin L, Hall WA, Jin N, Todhunter DA, Panoskaltis-Mortari A, Vallera DA. Targeting glioblastoma multiforme with an IL-13/diphtheria toxin fusion protein in vitro and in vivo in nude mice. Prot Engin. 2002;15:419–427.
- Debinski W, Gibo DM, Kealiher A, Puri RK. Novel anti-brain tumor cytotoxins specific for cancer cells. Nature Biotech. 1998;16:449–53. http://dx.doi.org/10.1038/nbt0598-449
- Mintz A, Gibo DM, Madhankumar AB, Debinski W. Molecular targeting with recombinant cytotoxins of interleukin-13 receptor alpha-2-expressing glioma. J Neuro-Oncol. 2003;64:117–23. http://dx.doi.org/10.1007/BF02700026
- Ulasov IV, Tyler MA, Han Y, Glasgow JN, Lesniak MS. Novel recombinant adenoviral vector that targets the interleukin-13 receptor alpha2 chain permits effective gene transfer to malignant glioma. Hum Gene Ther. 2007;18:118–29. http://dx.doi.org/10.1089/hum.2006.146
- Brown CE, Alizadeh D, Starr R, Weng L, Wagner JR, Naranjo A, et al. Regression of glioblastoma after chimeric antigen receptor T-cell therapy. N Engl J Med. 2016 Dec 29;375(26):2561–9. http://dx.doi.org/10.1056/NEJMoa1610497
- Balyasnikova IV, Wainwright DA, Solomaha E, Lee G, Han Y, Thaci B, Lesniak MS. Characterization and immunotherapeutic implications for a novel antibody targeting interleukin (IL)-13 receptor α2. J Biol Chem. 2012;287(36):30215–27. http://dx.doi.org/10.1074/jbc.M112.370015
- Kong S, Sengupta S, Tyler B, Bais AJ, Ma Q, Doucette S, et al. Suppression of human glioma xenografts with second-generation IL13R-specific chimeric antigen receptor-modified T cells. Clin Cancer Res. 2012 Nov 1;18(21):5949–60. http://dx.doi.org/10.1158/1078-0432.CCR-12-0319
- Okada H, Kalinski P, Ueda R, Hoji A, Kohanbash G, Donegan TE, et al. Induction of CD8+ T-cell responses against novel glioma-associated antigen peptides and clinical activity by vaccinations with {alpha}-type 1 polarized dendritic cells and polyinosinic-polycytidylic acid stabilized by lysine and carboxymethylcellulose in patients with recurrent malignant glioma. J Clin Oncol. 2011 Jan 20;29(3):330–6. http://dx.doi.org/10.1200/JCO.2010.30.7744
- Okada H, Kalinski P, Ueda R, Hoji A, Kohanbash G, Donegan TE, et al. Antigenic profiling of glioma cells to generate allogeneic vaccines or dendritic cell–based therapeutics. Clin Cancer Res. 2007;13:566–75. http://dx.doi.org/10.1158/1078-0432.CCR-06-1576
- Turtle CJ, Hudecek M, Jensen MC, Riddell SR. Engineered T cells for anti-cancer therapy. Curr Opin Immunol. 2012 Oct;24(5):633–9. http://dx.doi.org/10.1016/j.coi.2012.06.004
- Allen C, Paraskevakou G, Iankov I, Giannini C, Schroeder M, Sarkaria J, et al. Interleukin-13 displaying retargeted oncolytic measles virus strains have significant activity against gliomas with improved specificity. Mol Ther. 2008 Sep;16(9):1556–64. http://dx.doi.org/10.1038/mt.2008.152
- Wykosky J, Debinski W. The EphA2 receptor and ephrinA1 ligand in solid tumors: Function and therapeutic targeting. Mol Cancer Res. 2008;6:1795–806. http://dx.doi.org/10.1158/1541-7786.MCR-08-0244
- Hatano M, Eguchi J, Tatsumi T, Kuwashima N, Dusak JE, Kinch MS, et al. EphA2 as a glioma-associated antigen: A novel target for glioma vaccines. Neoplasia. 2005 Aug;7(8):717–22. http://dx.doi.org/10.1593/neo.05277
- Debinski W, Obiri NI, Pastan I, Puri RK. A novel chimeric protein composed of interleukin 13 and Pseudomonas exotoxin is highly cytotoxic to human carcinoma cells expressing receptors for interleukin 13 and interleukin 4. J Biol Chem. 1995;270:16775–80. http://dx.doi.org/10.1074/jbc.270.28.16775
- Debinski W, Obiri NI, Powers SK, Pastan I, Puri RK. Human glioma cells overexpress receptor for interleukin 13 and are extremely sensitive to a novel chimeric protein composed of interleukin 13 and Pseudomonas exotoxin. Clin Cancer Res. 1995;1:1253–8.
- Debinski W, Tatter SB. Convection-enhanced delivery for the treatment of brain tumors. Expert Rev Neurother. 2009;9:1519–27. http://dx.doi.org/10.1586/ern.09.99
- Ferluga S, Tomé CM, Herpai DM, D’Agostino R, Debinski W. Simultaneous targeting of Eph receptors in glioblastoma. Oncotarget. 2016 Sep 13;7(37):59860–76. http://dx.doi.org/10.18632/oncotarget.10978
- Hatano M, Kuwashima N, Tatsumi T, Dusak JE, Nishimura F, Reilly KM, et al. Vaccination with EphA2-derived T cell-epitopes promotes immunity against both EphA2-expressing and EphA2negative tumors. J Transl Med. 2004 Nov 24;2(1):40. http://dx.doi.org/10.1186/1479-5876-2-40
- Brown CE, Warden CD, Starr R, Deng X, Badie B, Yuan YC, et al. Glioma IL13Rα2 is associated with mesenchymal signature gene expression and poor patient prognosis. PLoS One. 2013 Oct 18;8(10):e77769. http://dx.doi.org/10.1371/journal.pone.0077769
- Brown CE, Starr R, Aguilar B, Shami AF, Martinez C, D’Apuzzo M, et al. Stem-like tumor-initiating cells isolated from IL-13Rα2 expressing gliomas are targeted and killed by IL13-zetakine-redirected T Cells. Clin Cancer Res. 2012;18:2199–209. http://dx.doi.org/10.1158/1078-0432.CCR-11-1669
- Brown CE, Starr R, Martinez C, Aguilar B, D’Apuzzo M, Todorov I, et al. Recognition and killing of brain tumor stem-like initiating cells by CD8+ cytolytic T cells. Cancer Res. 2009;69(23):8886–93. http://dx.doi.org/10.1158/0008-5472.CAN-09-2687
- Nguyen V, Conyers JM, Zhu D, Gibo DM, Dorsey JF, Debinski W, et al. IL-13Rα2-targeted therapy escapees: Biologic and therapeutic implications. Transl Oncol. 2011;4:390–400. http://dx.doi.org/10.1593/tlo.11175
- Binda E, Visioli A, Giani F, Lamorte G, Copetti M, Pitter KL, et al. The EphA2 receptor drives self-renewal and tumorigenicity in stem-like tumor-propagating cells from human glioblastomas. Cancer Cell. 2012;22(6):765–80. http://dx.doi.org/10.1016/j.ccr.2012.11.005
- Miao H, Gale NW, Guo H, Qian J, Petty A, Kaspar J, et al. EphA2 promotes infiltrative invasion of glioma stem cells in vivo through cross-talk with Akt and regulates stem cell properties. Oncogene. 2015 Jan 29;34(5):558–67. http://dx.doi.org/10.1038/onc.2013.590
- Day BW, Stringer BW, Al-Ejeh F, Ting MJ, Wilson J, Ensbey KS, et al. EphA3 maintains tumorigenicity and is a therapeutic target in glioblastoma multiforme. Cancer Cell. 2013;23(2):238–48. http://dx.doi.org/10.1016/j.ccr.2013.01.007
- Himanen JP, Chumley MJ, Lackmann M, Li C, Barton WA, Jeffrey PD, et al. Repelling class discrimination: Ephrin-A5 binds to and activates EphB2 receptor signaling. Nat Neurosci. 2004 May;7(5): 501–509. http://dx.doi.org/10.1038/nn1237
- Nakada M, Niska JA, Miyamori H, McDonough WS, Wu J, Sato H, Berens ME. The phosphorylation of EphB2 receptor regulates migration and invasion of human glioma cells. Cancer Res. 2004 May 1;64(9):3179–3185. http://dx.doi.org/10.1158/0008-5472.CAN-03-3667
- Hsi LC, Kundu S, Palomo J, Xu B, Ficco R, Vogelbaum MA, Cathcart MK. Silencing IL-13Rα2 promotes glioblastoma cell death via endogenous signaling. Mol Cancer Ther. 2011 Jul;10(7):1149–60. http://dx.doi.org/10.1158/1535-7163.MCT-10-1064
- Tu M, Wange W, Cai L, Zhu P, Gao Z, Zheng W. IL-13 receptor α2 stimulates human glioma cell growth and metastasis through the Src/PI3K/Akt/mTOR signaling pathway. Tumour Biol. 2016 Nov;37(11):14701–9. http://dx.doi.org/10.1007/s13277-016-5346-x
- Wykosky J, Gibo DM, Stanton C, Debinski W. EphA2 as a novel molecular marker and target in glioblastoma multiforme. Mol Cancer Res. 2005;3(10):541–51. http://dx.doi.org/10.1158/1541-7786.MCR-05-0056
- Wykosky J, Palma E, Gibo DM, Ringler S, Turner CP, Debinski W. Soluble monomeric ephrinA1 is released from tumor cells and is a functional ligand for the EphA2 receptor. Oncogene. 2008;27: 7260–73. http://dx.doi.org/10.1038/onc.2008.328
- Wykosky J, Gibo DM, Debinski W. A novel, potent, and specific ephrinA1-based cytotoxin against EphA2 receptor-expressing tumor cells. Mol Cancer Ther. 2007;6:3208–18. http://dx.doi.org/10.1158/1535-7163.MCT-07-0200
- Beckmann MP, Cerretti DP, Baum P, Vanden Bos T, James L, Farrah T, et al. Molecular characterization of a family of ligands for eph-related tyrosine kinase receptors. EMBO J. 1994;13:3757–62.
- Xu Q, Wilkinson DG. Eph-related receptors and their ligands: Mediators of contact dependent cell interactions. J Mol Med. 1997;75:576–86. http://dx.doi.org/10.1007/s001090050142
- Kullander K, Klein R. Mechanisms and functions of Eph and ephrin signalling. Nat Rev Mol. Cell Biol. 2002;3:475–86. http://dx.doi.org/10.1038/nrm856
- Pasquale EB. Eph receptor signalling casts a wide net on cell behaviour. Nat Rev Mol Cell Biol. 2005;6:462–75. http://dx.doi.org/10.1038/nrm1662
- Liu F, Park PJ, Lai W, Maher E, Chakravarti A, Durso L, et al. A genome-wide screen reveals functional gene clusters in the cancer genome and identifies EphA2 as a mitogen in glioblastoma. Cancer Res. 2006;66:10815–23. http://dx.doi.org/10.1158/0008-5472.CAN-06-1408
- Li X, Wang Y, Wang Y, Zhen H, Yang H, Fei Z, et al. Tumour Biol. 2007;28:165–72. http://dx.doi.org/10.1159/000103010
- Wang LF, Fokas E, Bieker M, Rose F, Rexin P, Zhu Y, et al. Increased expression of EphA2 correlates with adverse outcome in primary and recurrent glioblastoma multiforme patients. Oncol Rep. 2008;19:151–6. http://dx.doi.org/10.3892/or.19.1.151
- Beauchamp A, Debinski W. Ephs and ephrins in cancer: Ephrin-A1 signalling. Semin Cell Dev Biol. 2012 Feb;23(1):109–15. http://dx.doi.org/10.1016/j.semcdb.2011.10.019
- Debinski W, Wykosky J. Molecular targeting of IL-13R-2 and EphA2 receptor in GBM. In: van Meir E, editor. CNS cancer: Models, prognostic factors and targets. New York: Springer; 2009. p. 841–858.
- Debinski W. Drug cocktails for effective treatment of glioblastoma multiforme. Expert Rev Neurother. 2008;8(4):515–517. http://dx.doi.org/10.1586/14737175.8.4.515
- Li W, Graeber MB. The molecular profile of microglia under the influence of glioma. Neuro Oncol. 2012;14(8):958–78. http://dx.doi.org/10.1093/neuonc/nos116
- Chen R, Nishimura MC, Bumbaca SM, Kharbanda S, Forrest WF, Kasman IM, et al. A hierarchy of self-renewing tumor-initiating cell types in glioblastoma. Cancer Cell. 2010 Apr 13;17(4):362–75. http://dx.doi.org/10.1016/j.ccr.2009.12.049
- Laske DW, Youle RJ, Oldfield EH. Tumor regression with regional distribution of the targeted toxin TF-CRM107 in patients with malignant brain tumors. Nature Med. 1997;3:1362–8. http://dx.doi.org/10.1038/nm1297–1362
- Debinski W. Molecular targeting of brain tumors with cytotoxins. In: Lorberboum-Galski H, Lazarovici P, editors. Chimeric toxins. Reading, UK: Harwood Academic Publishers Reading, UK; 2002. p. 222–246.
- Debinski W. Local treatment of brain tumors with targeted chimera cytotoxic proteins. Cancer Invest. 2002;20:801–9. http://dx.doi.org/10.1081/CNV-120003545
- Wersall P, Ohlsson I, Biberfeld P, Collins V, von Krusenstjerna Larsson S, Mellstedt H, et al. Intratumoral infusion of the monoclonal antibody, mAb 425, against the epidermal-growth-factor receptor in patients with advanced malignant glioma. Cancer Immunol Immunother. 1997;44:157–64. http://dx.doi.org/10.1007/s002620050368
- Raghavan R, Brady ML, Rodriguez-Ponce MI, Hartlep A, Pedain C, Sampson JH. Convection-enhanced delivery of therapeutics for brain disease, and its optimization. Neurosurg Focus. 2006 Apr 15;20(4):E12. http://dx.doi.org/10.3171/foc.2006.20.4.7
- Yun J, Rothrock RJ, Canoll P, Bruce JN. Convection-enhanced delivery for targeted delivery of anti-glioma agents: The translational experience. J Drug Deliv. 2013;2013:107573.
- Vogelbaum MA, Iannotti CA. Convection-enhanced delivery of therapeutic agents into the brain. Handb Clin Neurol. 2012;104:355–62. http://dx.doi.org/10.1016/B978-0-444-52138-5.00023-2
- Chen PY, Ozawa T, Drummond DC, Kalra A, Fitzgerald JB, Kirpotin DB, et al. Comparing routes of delivery for nanoliposomal irinotecan shows superior anti-tumor activity of local administration in treating intracranial glioblastoma xenografts. Neuro Oncol. 2013 Feb;15(2):189–97. http://dx.doi.org/10.1093/neuonc/nos305
- Richardson RM, Kells AP, Martin AJ, Larson PS, Starr PA, Piferi PG, et al. Novel platform for MRI-guided convection-enhanced delivery of therapeutics: Preclinical validation in nonhuman primate brain. Stereotact Funct Neurosurg. 2011;89(3):141–51. http://dx.doi.org/10.1159/000323544
- Mehta AI, Choi BD, Raghavan R, Brady M, Friedman AH, Bigner DD, et al. Imaging of convection enhanced delivery of toxins in humans. Toxins (Basel). 2011 Mar;3(3):201–206. http://dx.doi.org/10.3390/toxins3030201
- Sampson JH, Brady M, Raghavan R, Mehta AI, Friedman AH, Reardon DA, et al. Colocalization of gadolinium-diethylene triamine pentaacetic acid with high-molecular-weight molecules after intracerebral convection-enhanced delivery in humans. Neurosurgery. 2011 Sep;69(3):668–76. http://dx.doi.org/10.1227/NEU.0b013e3182181ba8
- Debinski W, Tatter SB. Convection-enhanced delivery to achieve widespread distribution of viral vectors: Predicting clinical implementation. Curr Opin Mol Ther. 2010 Dec;12(6):647–53.
- Vogelbaum MA, Aghi MK. Convection-enhanced delivery for the treatment of glioblastoma. Neuro Oncol. 2015 Mar;17(Suppl 2):ii3–ii8. http://dx.doi.org/10.1093/neuonc/nou354
- Chen TC, Napolitano GR, Adell F, Schönthal AH, Shachar Y. Development of the metronomic biofeedback pump for leptomeningeal carcinomatosis: Technical note. J Neurosurg. 2015 Aug;123(2): 362–72. http://dx.doi.org/10.3171/2014.10.JNS14343
- Wainwright DA, Nigam P, Thaci B, Dey M, Lesniak MS. Recent developments on immunotherapy for brain cancer. Expert Opin Emerg Drugs. 2012 Jun;17(2):181–202. http://dx.doi.org/10.1517/14728214.2012.679929
- Wu A, Wei J, Kong LY, Wang Y, Priebe W, Qiao W, et al. Glioma cancer stem cells induce immunosuppressive macrophages/microglia. Neuro Oncol. 2010 Nov;12(11):1113–25. http://dx.doi.org/10.1093/neuonc/noq082
- Wainwright DA, Dey M, Chang A, Lesniak MS. Targeting tregs in malignant brain cancer: Overcoming IDO. Front Immunol. 2013 May 15;4:116. http://dx.doi.org/10.3389/fimmu.2013.00116
- Avril T, Vauleon E, Tanguy-Royer S, Mosser J, Quillien V. Mechanisms of immunomodulation in human glioblastoma. Immunotherapy. 2011 Apr;3(4 Suppl):42–4. http://dx.doi.org/10.2217/imt.11.39
- Ochiai H, Archer GE, Herndon JE 2nd, Kuan CT, Mitchell DA, Bigner DD, et al. EGFRvIIItargeted immunotoxin induces antitumor immunity that is inhibited in the absence of CD4+ and CD8+ T cells. Cancer Immunol Immunother. 2008 Jan;57(1):115–21. http://dx.doi.org/10.1007/s00262-007-0363-7
- Lang FF, Conrad C, Gomez-Manzano C, Tufaro F, Yung WKA, Sawaya R,et al. First-in-human Phase I clinical trial of oncolytic delta-24-RGD (DNX-2401) with biological endpoints: Implications for viroimmunotherapy. Neuro Oncol. 2014;16(Suppl 3):iii39. http://dx.doi.org/10.1093/neuonc/nou208.61
- Harrington KJ, Andtbacka RH, Collichio F, Downey G, Chen L, Szabo Z, et al. Efficacy and safety of talimogene laherparepvec versus granulocyte-macrophage colony-stimulating factor in patients with stage IIIB/C and IVM1a melanoma: Subanalysis of the Phase III OPTiM trial. Onco Targets Ther. 2016 Nov 16;9:7081–7093.
- Couzin-Frankel J. Breakthrough of the year 2013. Cancer immunotherapy. Science. 2013 Dec 20;342(6165):1432–3. http://dx.doi.org/10.1126/science.342.6165.1432
- Hu-Lieskovan S, Robert L, Homet Moreno B, Ribas A. Combining targeted therapy with immunotherapy in BRAF-mutant melanoma: Promise and challenges. J Clin Oncol. 2014 Jul 20;32(21): 2248–54. http://dx.doi.org/10.1200/JCO.2013.52.1377
- Kunwar S, Prados MD, Chang SM, Berger MS, Lang FF, Piepmeier JM, et al. Direct intracerebral delivery of cintredekin besudotox (IL13-PE38QQR) in recurrent malignant glioma: A report by the Cintredekin Besudotox Intraparenchymal Study Group. J Clin Oncol. 2007 Mar 1;25(7):837–44. http://dx.doi.org/10.1200/JCO.2006.08.1117
- Candolfi M, Xiong W, Yagiz K, Liu C, Muhammad AK, Puntel M, et al. Gene therapy-mediated delivery of targeted cytotoxins for glioma therapeutics. Proc Natl Acad Sci. 2010;107:20021–6. http://dx.doi.org/10.1073/pnas.1008261107
- Thompson J, Debinski W. Mutants of interleukin 13 with altered reactivity toward IL13 receptors. J Biol Chem. 1999;274:29944–50. http://dx.doi.org/10.1074/jbc.274.42.29944
- Madhankumar AB, Mintz A, Debinski W. Alanine scanning mutagenesis of a-helix D segment of interleukin-13 reveals new functionally important residues of the cytokine. J Biol Chem. 2002;277: 43194–205. http://dx.doi.org/10.1074/jbc.M205047200
- Madhankumar AB, Mintz A, Debinski W. Interleukin 13 mutants of enhanced avidity toward the glioma-associated receptor, IL13Ralpha2. Neoplasia. 2004 Jan–Feb;6(1):15–22. http://dx.doi.org/10.1016/S1476-5586(04)80049-6
- Sonawane P, Choi YA, Pandya H, Herpai DM, Fokt I, Priebe W, et al. Novel molecular multilevel targeted antitumor agents. Cancer Transl Med. 2017;3(3):69–79.